Differentiation of the brain vasculature: the answer came blowing by the Wnt
Journal of Angiogenesis Research. 2010;
Received: 20 November 2009 | Accepted: 14 January 2010 | Published: 14 January 2010
Vascular Cell ISSN: 2045-824X
Abstract
Vascularization of the vertebrate brain takes place during embryonic development from a preformed perineural vascular plexus. As a consequence of the intimate contact with neuroectodermal cells the vessels, which are entering the brain exclusively via sprouting angiogenesis, acquire and maintain unique barrier properties known as the blood-brain barrier (BBB). The endothelial BBB depends upon the close association of endothelial cells with pericytes, astrocytes, neurons and microglia, which are summarized in the term neuro-vascular unit. Although it is known since decades that the CNS tissue provides the cues for BBB induction and differentiation in endothelial cells, the molecular mechanism remained obscure.
Only recently, the canonical Wnt/β-catenin pathway and the Wnt7a/7b growth factors have been implicated in brain angiogenesis on the one hand and in BBB induction on the other. This breakthrough in understanding the differentiation of the brain vasculature prompted us to review these findings embedded in the emerging concepts of Wnt signaling in the vasculature. In particular, interactions with other pathways that are crucial for vascular development such as VEGF, Notch, angiopoietins and
Introduction
In vertebrate ontogenesis, blood vessels arise through differentiation of mesodermal hemangioblasts into endothelial cells (ECs), which assemble into so-called blood islands of the extraembryonic yolk sac and into the aortic primordia of the embryo proper [1]. The first ECs form a primitive vascular network in a process defined as vasculogenesis with subsequent growth and remodeling of preformed vessels known as angiogenesis [2]. In fact, most organs are vascularized by initial vasculogenesis subsequently followed by angiogenic sprouting.
The central nervous system (CNS) however, is exclusively vascularized by angiogenesis starting at embryonic day 9 in rodents from the perineural vascular plexus (PVP), covering the entire surface of the neural tube [2]. In the adult, vessels will supply the brain with oxygen and glucose and they assure that metabolic end products are removed to maintain tissue homeostasis. This is of upmost importance as the function of neurons and glia depends on ion-based concentration gradients established in brain fluids, i.e. cerebrospinal fluid (CSF), providing a unique milieu for electrical nerve cell communication. Consequently, the brain is protected from the free diffusion between blood plasma and the interstitium by a vascular blood-brain barrier (BBB) in order to maintain CSF homeostasis.
The mature BBB consists of a complex cellular system in which capillaries are regularly covered by a high number of pericytes embedded in a common basal membrane and by astrocytic endfeet. The circumference of brain capillaries is lined by a single endothelial cell connected with itself and neighboring endothelial cells by complex tight junctions (TJs), intermingled with non-occluding adherens junctions (AJs) [3]. AJs are formed by the endothelial specific integral membrane protein VE-cadherin, which is linked to the cytoskeleton via catenins. Endothelial expression and localization of β-catenin, γ-catenin and p120ctn have been described to be crucial for the functional state of AJs including those of brain ECs (for review see [4]) (Figure 1). TJs in the CNS of mice and men are formed by occludin, as well as by members of the claudin family of transmembrane proteins [5]. In particular the endothelial specific claudin-5, which is also present in non-brain ECs, and claudin-3 were shown to localize to endothelial TJs in the CNS [6]. Moreover, claudin-1 and claudin-12 are described in brain ECs but their role is still unclear [6, 7] (Figure 1). The complex TJs between brain ECs establish a high electrical resistance across the endothelial barrier (about 2000Ω × cm2) that is the hallmark of the BBB [8].
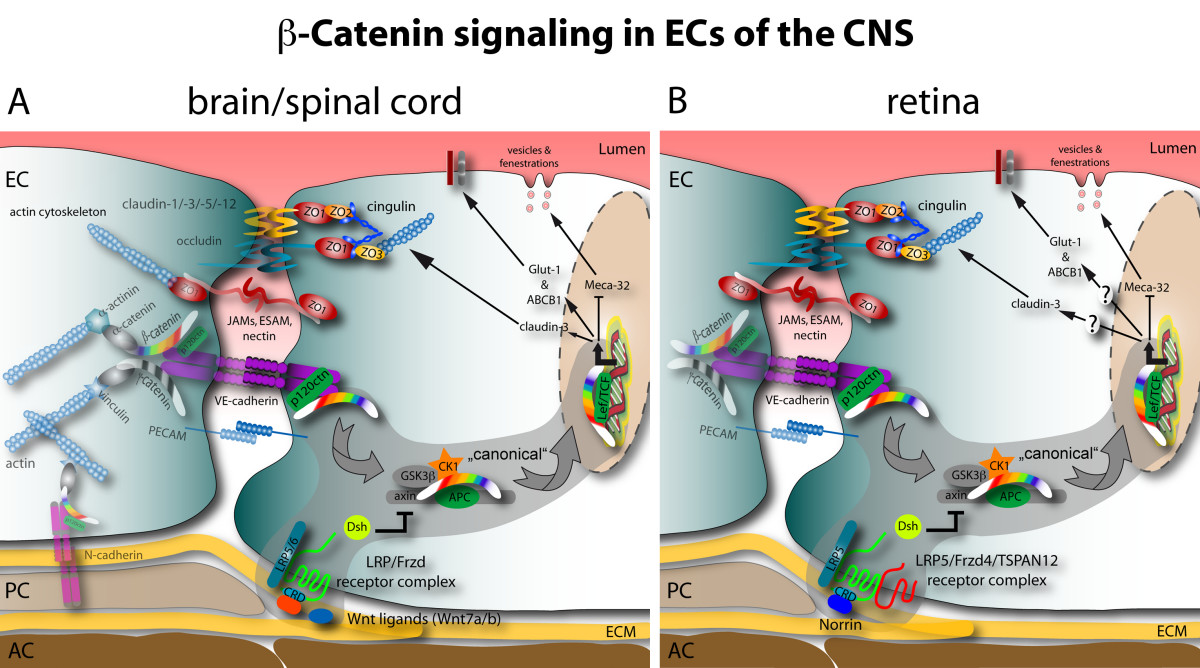
Figure 1
Figure 1 caption
Schematic junctional organization and β-catenin signaling in CNS ECs: comparison of brain and retina. A In the brain and spinal cord mainly Wnt7a and Wnt7b growth factors act on an uncharacterized Fzd-LRP receptor complex to elicit β-catenin target gene transcription. The positively regulated target genes identified so far are the TJ protein claudin-3, Glut-1 and ABCB1/MDR1. Conversely, the expression of Meca-32/Plvap becomes suppressed by β-catenin transcription via an unknown mechanism. B in the retina Norrin is the predominant ligand activating β-catenin signaling downstream of the Fzd4-LRP5-TSPAN12 receptor complex. So far only the repression of Meca-32/Plvap was identified as target of this signaling. To date the effect of Norrin signaling on claudin-3, Glut-1 or other barrier-related genes is not know. See the grey supported area and arrows for the "canonical" Wnt pathway.
As ECs start to form an elaborate network of TJs during BBB differentiation, they start also to express selective transporters, such as glucose transporter type1 (Glut-1), members of the ATP binding cassette (ABC) transporter family (ABCB1/P-glycoprotein/MDR1 and ABCG2), and enzymes which together establish the complex phenotype of the BBB (for review see [9]).
In contrast to the genes, which become upregulated in brain endothelia during BBB maturation, the panendothelial cell antigen MECA-32 (also known as plasmalemma vesicle associated protein 1, Plvap1) becomes specifically down regulated [10]. As a consequence, the MECA-32 antigen is absent on the mature cerebral endothelium, whereas it remains present on vessels outside the CNS and on capillaries within the circumventricular organs (CVOs) (Figure 1).
Driven by the question what makes endothelial cells grow into the neuroectoderm, in the 1980s Werner Risau✝ hypothesized that soluble factors produced by the brain and recognized by specific receptors on ECs of the PVP are responsible for brain angiogenesis. Although the initial candidates aFGF/bFGF were potent inducers of EC proliferation
One major drawback for understanding brain angiogenesis is that these mechanisms also apply outside the CNS. Therefore, it seems unlikely that they are specifically involved in BBB differentiation creating highly specialized ECs.
The motivation for this review is drawn from recent reports indicating that in particular the Wnt ligands Wnt 7a/b, signaling via the Wnt/β-catenin pathway, could act as CNS-specific morphogens regulating particularly steps of angiogenesis and vascular differentiation [20–22].
The players in the Wnt pathway
Wnt signaling regulates various biological processes on a cellular level such as proliferation, apoptosis, polarity and differentiation as well as pluripotency of stem cells. These functions have consequences on a tissue or organ level, influencing axis determination, segmentation as well as branching of tubular structures [23]. Wnts are a family of 19 secreted glycoproteins that primarily signal through seven-pass transmembrane receptors of the frizzled family (Fzd) [23]. Wnt proteins undergo extensive post-translational modification, such as glycosylation and palmitoylation, which influence solubility and diffusability of the proteins, and their accumulation in the extracellular matrix [24, 25]. The term "Wnt signaling" comprises at least three related pathways (see Figure 2), of which the best-characterized one is the "canonical" or β-catenin (
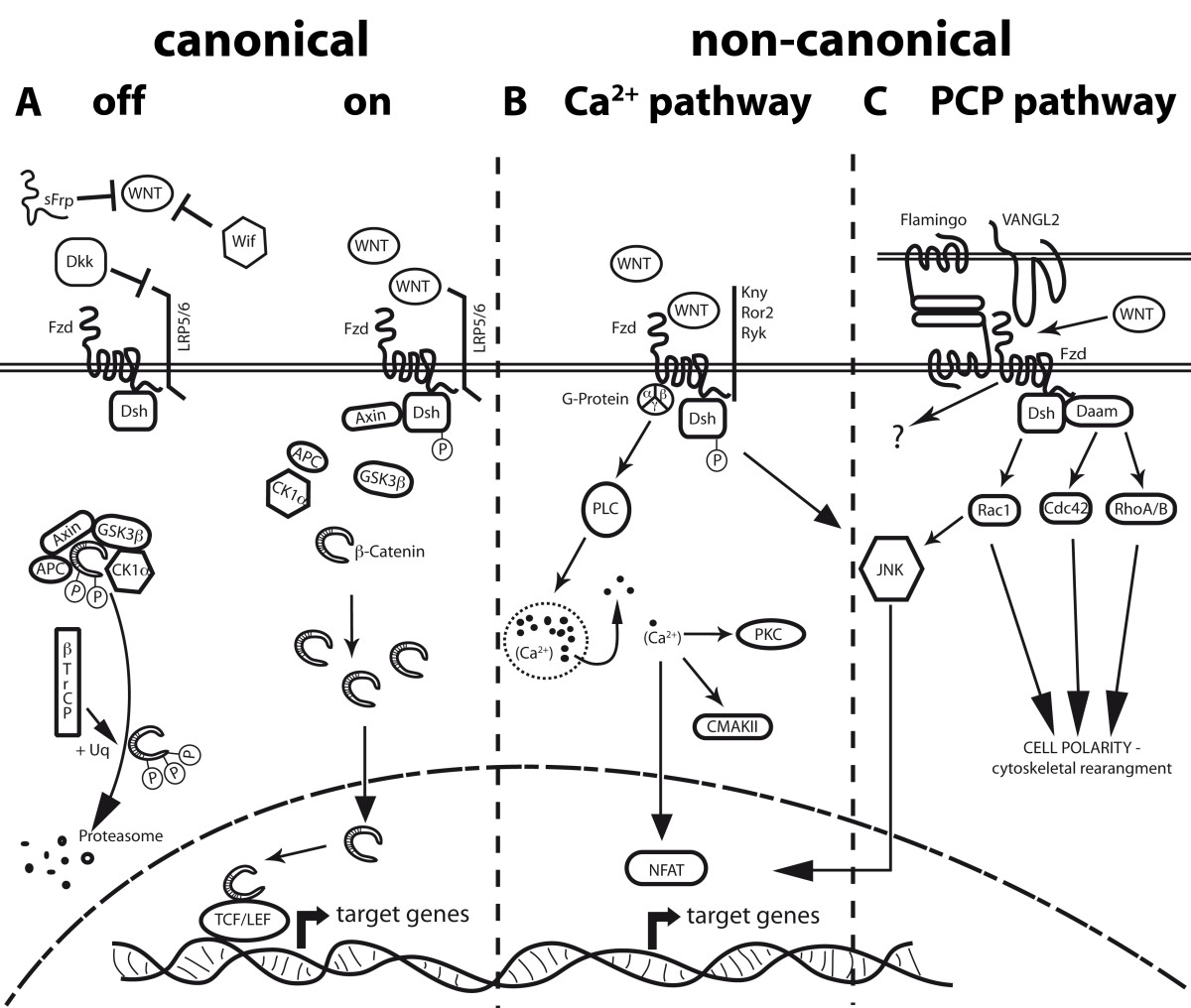
Figure 2
Figure 2 caption
Scheme of known Wnt pathways. A Canonical Wnt/β-catenin pathway. In the "off-state" no Wnt proteins are present or are inhibited by factors like WIF, sFRPs and Dkk. Cytosolic β-catenin is targeted to proteolytic degradation through phosphorylation by the APC-Axin-GSK3β-CK1á complex and ubiquitination by the βTrCP-dependent E3 ubiquitin ligase. In the "on-state" stimulation of Fzd receptors and their co-receptors Lrp5/6 by Wnt ligands, leads to recruitment of Dvl and Axin to Fzd, thereby inhibiting the degradation complex. Consequently, β-catenin accumulates in the cytoplasm and enters the nucleus, activating target gene transcription through association with Lef1/TCF. B Non-canonical Wnt/Ca pathway. Interaction of non-canonical Wnt ligands with Fzd receptors can lead to G-Protein mediated phosphorylation of Dsh, thereby activating PLC and increasing intracellular calcium levels. These will activate CAMKII and PKC, as well as the transcription factor NFAT. Additionally, Fzd receptors in association with Kny, Ror2 or Ryk receptors can activate JNK, promoting target gene expression through AP-1. C Non-canonical Wnt/PCP pathway. This pathway is characterized by an asymmetric distribution of Fzds, the cadherin Flamingo and VANGL2, resulting in cell polarization. Wnt signaling promoted by either Wnt or an interaction of Fzd with VANGL2 activates RhoA/B, Cdc42 or Rac1. Dsh activates Rac1, while RhoA/B and Cdc42 need the participation of Daam1 downstream of Dsh. Rac1 can also activate JNK, resulting in the NFAT pathway. All three down stream pathways are key players in cytoskeletal rearrangement and cellular polarity. The signaling of the Flamingo/Fzd interaction is still obscure.
The non-canonical pathways, which are defined by the independence of β-catenin as a central signaling molecule, are an assembly of by now loosely separable pathways. Wnt5a, Wnt4 and Wnt11 are considered to be prototype non-canonical Wnts, which do not induce axis duplication in Xenopus. Instead, non-canonical Wnts may induce Ca2+ release and activation of the protein kinase C (PKC) or Ca2+/calmodulin-dependent protein kinase (CamkII), both summarized as the Ca2+-pathway (Figure 2B). Alternatively, non-canonical Wnts can activate Rac, Cdc42, RhoA/B and downstream c-Jun N-terminal kinase (JNK) or RAB4, known as the planar cell polarity pathway (PCP). The latter has been identified in Drosophila, but is also present in vertebrate organisms and plays a crucial role in cellular convergence and extension movements during organogenesis [30]. PCP can also be activated/modulated by non-Wnt related, membrane bound Fzd ligands such as
Moreover, secreted antagonists, including Dickkopf homologs (Dkk1-4), secreted frizzled-related protein families (sFRPs, 1-5), and atypical Wnt receptors, such as the receptor-like tyrosine kinase (Ryk) and receptor tyrosine kinase-like orphan receptors (Ror), can regulate the Wnt signaling output (see Figure 2) [32]. Furthermore, Fzd ligands, which are structurally unrelated to Wnts, such as the Norrie disease pseudoglioma protein (Nrp, Norrin), have been described to activate β-catenin transcriptional activity particularly in the vascular system and are discussed in detail in the following chapters [33].
Wnt pathway in vascular beds of the central nervous system
So far the Wnt/β-catenin pathway has been reported to be involved in various aspects of EC biology and in angiogenesis, such as proliferation and vessel assembly. The reader is referred to the following review articles comprehensively summarizing the general role of the Wnt pathway during developmental vasculo- and angiogenesis (for review see [34–36]).
Endothelial Wnt pathway in CNS pathologies
Since Wnt signaling was identified to play a major role in vascular development and differentiation, it is of particular interest to understand its involvement in pathological angiogenesis in which hypoxia is known to be one of the major driving forces.
Tissue hypoxia takes also place in tumors and is described as a prerequisite for the angiogenic switch of various cancers, leading to the massive expression of VEGF-A by hypoxic tumor cells [71]. The most frequent and most malignant primary brain tumors of adulthood are WHO grade IV astrocytoma (glioblastoma), which are known to be highly hypoxic and extensively vascularized [72]. Recent reports suggest that β-catenin signaling in glioma cells participates in tumor cell progression and invasion [73–75]. This effect is, at least partially, mediated by the epidermal growth factor receptor (EGFR), which activates ERK leading to the dissociation of α- and β-catenin, thereby promoting β-catenin transactivation [73]. To which extend glioblastoma cells express Wnt growth factors, leading to autocrine and/or paracrine signaling to the vascular compartment, as it is known from VEGF, is poorly known.
Nevertheless, glioblastoma tumor vessels were demonstrated to regularly show nuclear β-catenin in human samples and in an N-ethyl-N-nitrosourea-induced rat glioma model [76, 77]. The specific function of endothelial β-catenin signaling in glioblastoma however has not been determined so far.
After stroke, independent of its etiology from ischemic or hemorrhagic insult, BBB integrity is focally abolished and during glia scar formation, inflammation and neo-angiogenesis occurs [78]. Lithium chloride (LiCl), which is a widely used inhibitor of GSK3β, was frequently applied to treat bipolar mood disorders. More recently, LiCl was implicated in the reduction of infarct volume in a rat model for stroke [79]. After middle cerebral artery occlusion (MCAO), LiCl had a beneficial effect on the survival of neurons up to 3 h after onset of stroke, but the effect on EC has not been determined. Interestingly, Guo and co-workers have shown that LiCl upregulates VEGF-A in human brain microvascular ECs and astrocytes
Finally, the familial form of Alzheimer's disease (AD), in which the presenilin-1 (
Important clinical insight into the involvement of Wnt signaling in vascular pathogenesis came from studies of the human disorders Norrie disease (ND), Coat's disease and familial exudative vitreoretinopathy (FEVR) as mentioned above [84]. FEVR has been linked to 4 different loci, EVR-1 to 4, three of which encode for Norrin, Fzd4 and Lrp5 [46].
Norrie disease is caused by a X-linked recessive mutation of Norrie disease pseudoglioma gene (NDP), coding for Norrin/Norrie disease protein (Ndp) [85, 86]. The mouse Ndp KO displays a lack of deep retinal capillary networks and subsequent retinal hypoxia, which is accompanied by an increased expression of the angiogenic factors VEGF-A, β3 integrin and Tie1 [45]. Norrie patients suffer from blindness of both eyes at birth or soon after, leukocoria and cateract formation. Additionally, they develop sensorineural hearing loss, impaired motor skills and mental retardation caused by vascular defects in the inner ear and the cerebellum (reviewed in [87]).
In contrast to NDP mutation, Fzd4 mutations are autosomal dominant and those of Lrp5 are autosomal recessive, but phenotypes are largely overlapping.
With the identification of TSPAN12 as a modulator of Norrin signaling that genetically and functionally interacts with Fzd4, another gene could potentially be mutated in the FEVR family of diseases, although no mutation has been discovered in humans so far [52].
Conclusions
The underlying mechanisms for CNS vascularization in general and for endothelial barrier differentiation in particular have been obscure for about one century, since Paul Ehrlich and Edwin Goldmann established the concept of the BBB. The recent identification of the Wnt/β-catenin pathway as a driving force for brain angiogenesis and BBB differentiation has shed first light onto this burning question. Although Wnt7a/7b were confirmed to be the major ligands activating β-catenin signaling in brain and spinal cord ECs, it remains elusive if exactly the same molecular pathway operates in the entire CNS to induce the BBB phenotype in ECs. This hypothesis is challenged in the retina, in which Norrin via Fzd4-Lrp5-TSPAN12 appears to be the predominant activator of β-catenin signaling in ECs. The finding that ECs can express the majority of Wnt pathway related genes
Furthermore, Wnt signals in the brain vasculature will integrate with other pathways such as Notch, FGF, TGFβ and
Acknowledgements
I would like to thank Fabian Schneider for support in figure preparation and proof reading. Cathrin Czupalla, Nicole Ziegler and Marco Reis for proof reading.
The authors are supported by the Deutsche Forschungsgemeinschaft (SFB/TR23, Excellence Cluster Cardio-Pulmonary System, ECCPS). European Union Health FP7. I apologize to authors of relevant work not cited due to space restrictions.
Authors’ original submitted files for images
Below are the links to the authors’ original submitted files for images.
Authors’ original file for figure 1
Authors’ original file for figure 2
References
- Mechanisms of endothelial differentiation in embryonic vasculogenesis. Arterioscler Thromb Vasc Biol. 2005;25:2246-2254.
- Mechanisms of angiogenesis. Nature. 1997;386:671-674.
- Immunohistochemical localization of adherens junction components in blood-brain barrier microvessels of the rat. J Cell Sci. 1993;104(Pt 3):773-782.
- The multiple languages of endothelial cell-to-cell communication. Arterioscler Thromb Vasc Biol. 2006;26:1431-1438.
- Brain endothelial cells and the glio-vascular complex. Cell Tissue Res. 2008.
- Localization of claudin-3 in tight junctions of the blood-brain barrier is selectively lost during experimental autoimmune encephalomyelitis and human glioblastoma multiforme. Acta Neuropathol. 2003;105:586-592.
- Size-selective loosening of the blood-brain barrier in claudin-5-deficient mice. J Cell Biol. 2003;161:653-660.
- Electrical resistance of brain microvascular endothelium. Brain Res. 1982;241:49-55.
- Development of the blood-brain barrier. Cell Tissue Res. 2003;314:119-129.
- Novel mouse endothelial cell surface marker is suppressed during differentiation of the blood brain barrier. Dev Dyn. 1995;202:325-332.
- Endothelial cell growth factors in embryonic and adult chick brain are related to human acidic fibroblast growth factor. Embo J. 1988;7:959-962.
- Vascular physiology. A family of angiogenic peptides. Nature. 1987;329:671-672.
- A heparin-binding angiogenic protein--basic fibroblast growth factor--is stored within basement membrane. Am J Pathol. 1988;130:393-400.
- Basic fibroblast growth factor (FGF) in the central nervous system: identification of specific loci of basic FGF expression in the rat brain. Growth Factors. 1989;2:21-29.
- Molecular cloning and developmental expression of rat fibroblast growth factor receptor 3. Histochem Cell Biol. 2001;115:147-155.
- Differentiating and mature neurons express the acidic fibroblast growth factor gene during chick neural development. Development. 1991;111:1143-1154.
- Development of the blood-brain barrier. In New Concepts of a blood-brain barrier. 1995.:11-31.
- The angiopoietins: Yin and Yang in angiogenesis. Curr Top Microbiol Immunol. 1999;237:173-185.
- Pericyte loss and microaneurysm formation in PDGF-B-deficient mice. Science. 1997;277:242-245.
- Wnt/beta-catenin signaling is required for CNS, but not non-CNS, angiogenesis. Proc Natl Acad Sci USA. 2009;106:641-646.
- Wnt/beta-Catenin signaling controls development of the blood-brain barrier. J Cell Biol. 2008;183:409-417.
- Canonical Wnt signaling regulates organ-specific assembly and differentiation of CNS vasculature. Science. 2008;322:1247-1250.
- Wnt/beta-catenin signaling in development and disease. Cell. 2006;127:469-480.
- Wnt family proteins are secreted and associated with the cell surface. Mol Biol Cell. 1993;4:1267-1275.
- Wnt proteins are lipid-modified and can act as stem cell growth factors. Nature. 2003;423:448-452.
- Control of beta-catenin phosphorylation/degradation by a dual-kinase mechanism. Cell. 2002;108:837-847.
- A ubiquitin ligase complex essential for the NF-kappaB, Wnt/Wingless, and Hedgehog signaling pathways. Genes Dev. 1999;13:505-510.
- A Wnt survival guide: from flies to human disease. J Invest Dermatol. 2009;129:1614-1627.
- The 'Marx' of Groucho on development and disease. Trends Cell Biol. 2007;17:353-361.
- Convergent extension: the molecular control of polarized cell movement during embryonic development. Dev Cell. 2002;2:695-706.
- Closing in on vertebrate planar polarity. Nat Cell Biol. 2004;6:687-689.
- The receptor tyrosine kinase Ror2 is involved in non-canonical Wnt5a/JNK signalling pathway. Genes Cells. 2003;8:645-654.
- Wnt signaling: Ig-norrin the dogma. Curr Biol. 2004;14:R436-437.
- Wnt/Frizzled signaling in angiogenesis. Angiogenesis. 2008;11:63-69.
- Wnt/Frizzled signaling in the vasculature: new angiogenic factors in sight. Physiology (Bethesda, Md). 2006;21:181-188.
- Vascular morphogenesis: a Wnt for every vessel?. Curr Opin Genet Dev. 2009;19:476-483.
- The conditional inactivation of the beta-catenin gene in endothelial cells causes a defective vascular pattern and increased vascular fragility. J Cell Biol. 2003;162:1111-1122.
- Beta-catenin is required for endothelial-mesenchymal transformation during heart cushion development in the mouse. J Cell Biol. 2004;166:359-367.
- Radial glial interaction with cerebral germinal matrix capillaries in the fetal baboon. Exp Neurol. 1992;118:126-132.
- Developing nervous tissue induces formation of blood-brain barrier characteristics in invading endothelial cells: a study using quail--chick transplantation chimeras. Dev Biol. 1981;84:183-192.
- Astrocyte-endothelial interactions at the blood-brain barrier. Nat Rev Neurosci. 2006;7:41-53.
- Nrarp coordinates endothelial Notch and Wnt signaling to control vessel density in angiogenesis. Dev Cell. 2009;16:70-82.
- Mice null for Frizzled4 (Fzd4-/-) are infertile and exhibit impaired corpora lutea formation and function. Biol Reprod. 2005;73:1135-1146.
- Mutant frizzled-4 disrupts retinal angiogenesis in familial exudative vitreoretinopathy. Nat Genet. 2002;32:326-330.
- Role of the Norrie disease pseudoglioma gene in sprouting angiogenesis during development of the retinal vasculature. Invest Ophthalmol Vis Sci. 2005;46:3372-3382.
- Mutations in LRP5 or FZD4 underlie the common familial exudative vitreoretinopathy locus on chromosome 11q. Am J Hum Genet. 2004;74:721-730.
- Vascular development in the retina and inner ear: control by Norrin and Frizzled-4, a high-affinity ligand-receptor pair. Cell. 2004;116:883-895.
- Cbfa1-independent decrease in osteoblast proliferation, osteopenia, and persistent embryonic eye vascularization in mice deficient in Lrp5, a Wnt coreceptor. J Cell Biol. 2002;157:303-314.
- A model for familial exudative vitreoretinopathy caused by LPR5 mutations. Hum Mol Genet. 2008;17:1605-1612.
- Obligatory participation of macrophages in an angiopoietin 2-mediated cell death switch. Development. 2007;134:4449-4458.
- WNT7b mediates macrophage-induced programmed cell death in patterning of the vasculature. Nature. 2005;437:417-421.
- TSPAN12 regulates retinal vascular development by promoting Norrin- but not Wnt-induced FZD4/beta-catenin signaling. Cell. 2009;139:299-311.
- Vascular changes in the cerebellum of Norrin/Ndph knockout mice correlate with high expression of Norrin and Frizzled-4. Eur J Neurosci. 2008;27:2619-2628.
- Activation of beta-catenin signalling by GSK-3 inhibition increases p-glycoprotein expression in brain endothelial cells. J Neurochem. 2008;106:1855-1865.
- Regulation of vascular endothelial growth factor by the Wnt and K-ras pathways in colonic neoplasia. Cancer Res. 2001;61:6050-6054.
- Glycogen-Synthase Kinase3beta/beta-catenin axis promotes angiogenesis through activation of vascular endothelial growth factor signaling in endothelial cells. Circ Res. 2005;96:308-318.
- Phosphatidylinositol 3-kinase/Akt stimulates androgen pathway through GSK3beta inhibition and nuclear beta-catenin accumulation. J Biol Chem. 2002;277:30935-30941.
- Angiogenesis: a team effort coordinated by notch. Dev Cell. 2009;16:196-208.
- Jagged1 is the pathological link between Wnt and Notch pathways in colorectal cancer. Proc Natl Acad Sci USA. 2009;106:6315-6320.
- Jagged 1 is a beta-catenin target gene required for ectopic hair follicle formation in adult epidermis. Development. 2006;133:4427-4438.
- The morphogen Sonic hedgehog is an indirect angiogenic agent upregulating two families of angiogenic growth factors. Nat Med. 2001;7:706-711.
- Angiogenesis within the developing mouse neural tube is dependent on sonic hedgehog signaling: possible roles of motor neurons. Genes Cells. 2005;10:595-604.
- Inhibitory Gli3 activity negatively regulates Wnt/beta-catenin signaling. Curr Biol. 2007;17:545-550.
- The role of glycogen synthase kinase-3beta in normal haematopoiesis, angiogenesis and leukaemia. Curr Med Chem. 2008;15:1493-1499.
- The AKT/IkappaB kinase pathway promotes angiogenic/metastatic gene expression in colorectal cancer by activating nuclear factor-kappaB and beta-catenin. Oncogene. 2005;24:1021-1031.
- Crosstalk between NF-kappaB and beta-catenin pathways in bacterial-colonized intestinal epithelial cells. Am J Physiol Gastrointest Liver Physiol. 2005;289:G129-137.
- Interplay between VHL/HIF1alpha and Wnt/beta-catenin pathways during colorectal tumorigenesis. Oncogene. 2006;25:3065-3070.
- Interaction between beta-catenin and HIF-1 promotes cellular adaptation to hypoxia. Nat Cell Biol. 2007;9:210-217.
- SSeCKS regulates angiogenesis and tight junction formation in blood-brain barrier. Nat Med. 2003;9:900-906.
- Ligand-specific control of src-suppressed C kinase substrate gene expression. Biochem Biophys Res Commun. 2002;297:1112-1120.
- Up-regulation of vascular endothelial growth factor expression in a rat glioma is conferred by two distinct hypoxia-driven mechanisms. Cancer Res. 1997;57:3860-3864.
- VEGF in brain tumors. J Neurooncol. 2000;50:109-120.
- EGF-Induced ERK Activation Promotes CK2-Mediated Disassociation of alpha-Catenin from beta-Catenin and Transactivation of beta-Catenin. Mol Cell. 2009;36:547-559.
- Activation of Wnt/beta-catenin/Tcf signaling pathway in human astrocytomas. Neurochem Int. 2009;55:307-317.
- Wnt/beta-catenin/Tcf signaling pathway activation in malignant progression of rat gliomas induced by transplacental N-ethyl-N-nitrosourea exposure. Neurochem Res. 2009;34:1278-1288.
- Immunohistochemical analysis of beta-catenin in N-ethyl-N-nitrosourea- induced rat gliomas: implications in regulation of angiogenesis. Neurol Res. 2000;22:527-532.
- Differential expression of beta-catenin in human glioblastoma multiforme and normal brain tissue. Neurol Res. 2000;22:650-656.
- The blood-brain barrier in brain homeostasis and neurological diseases. Biochim Biophys Acta. 2008.
- Postinsult treatment with lithium reduces brain damage and facilitates neurological recovery in a rat ischemia/reperfusion model. Proc Natl Acad Sci USA. 2003;100:6210-6215.
- Lithium upregulates vascular endothelial growth factor in brain endothelial cells and astrocytes. Stroke. 2009;40:652-655.
- Functional MRI of delayed chronic lithium treatment in rat focal cerebral ischemia. Stroke. 2008;39:439-447.
- Destabilization of beta-catenin by mutations in presenilin-1 potentiates neuronal apoptosis. Nature. 1998;395:698-702.
- Amyloid-beta binds to the extracellular cysteine-rich domain of Frizzled and inhibits Wnt/beta-catenin signaling. J Biol Chem. 2008;283:9359-9368.
- The Wnt signaling pathway in familial exudative vitreoretinopathy and Norrie disease. Semin Ophthalmol. 2007;22:211-217.
- Isolation of a candidate gene for Norrie disease by positional cloning. Nat Genet. 1992;2:84-.
- Isolation and characterization of a candidate gene for Norrie disease. Nat Genet. 1992;1:204-208.
- Molecular dissection of Norrie disease. Acta Anat (Basel). 1998;162:95-100.