Targeting lymphatic vessel functions through tyrosine kinases
Journal of Angiogenesis Research. 2010;
Received: 21 June 2010 | Accepted: 11 August 2010 | Published: 11 August 2010
Vascular Cell ISSN: 2045-824X
Abstract
The lymphatic vascular system is actively involved in tissue fluid homeostasis, immune surveillance and fatty acid transport. Pathological conditions can arise from injury to the lymphatics, or they can be recruited in the context of cancer to facilitate metastasis. Protein tyrosine kinases are central players in signal transduction networks and regulation of cell behavior. In the lymphatic endothelium, tyrosine kinases are involved in processes such as the maintenance of existing lymphatic vessels, growth and maturation of new vessels and modulation of their identity and function. As such, they are attractive targets for both existing inhibitors and the development of new inhibitors which affect lymphangiogenesis in pathological states such as cancer. RNAi screening provides an opportunity to identify the functional role of tyrosine kinases in the lymphatics. This review will discuss the role of tyrosine kinases in lymphatic biology and the potential use of inhibitors for anti-lymphangiogenic therapy.
Introduction
A number of human diseases have been linked to abnormal or defective lymphatic vessels [1]. While the theory of anti-angiogenesis therapy has been extensively studied [2], the concept of targeting lymphangiogenesis to gain a therapeutic advantage in human disease is only a recent development [1]. Advances in our understanding of the molecular signaling pathways that control lymphatic vessel formation therefore provide an opportunity to explore the value of inhibiting these processes.
A good example of this is cancer biology, where the spread of tumor cells appears highly dependent on the vessels of the lymphatic system and the protein factors which drive their growth and differentiation [3]. As a consequence, therapeutic options which target these cellular pathways may provide a means to prevent growth or metastasis from the primary tumor. Therapeutics may be either anti-lymphatic (targeting functions of the existing vessels) and/or anti-lymphangiogenic (targeting the generation of new lymphatic vessels). An understanding of the key signaling components and cellular processes that are critical for lymphatic vessel function and growth is essential to enable the rational design of effective inhibitors.
One family of molecules, the protein tyrosine kinases, are known to be key drivers of angiogenesis [4], and studies have shown they also play a pivotal role in lymphatic biology/lymphangiogenesis [5]. In this review we explore the potential for this family of molecules to be used as targets for anti-lymphatic/anti-lymphangiogenesis and the ways in which we can gain insight into how these family members might contribute to key signaling pathways within the lymphatic endothelium.
The lymphatic system in health and disease
While blood vessels carry oxygenated blood and nutrients to cells within the body, the lymphatic vessels act to maintain fluid homeostasis by draining excess fluid from the tissues, as well as contributing to immune surveillance and fatty acid transport. Fluid and cells released by the blood vessels are returned to the circulation via protein-rich lymph fluid that is drained by blind-ended capillaries in the superficial dermis. This is fed into the deeper, larger caliber lymphatic collecting vessels via lymph nodes and the thoracic duct and back to the circulation. All of these vessels have a specialized lining of endothelial cells. Both blood and lymphatic endothelial cells originate from common developmental precursors. Yet, it is now clear that the lymphatic endothelial cells differ in their molecular and physiological behavior to the "classical" blood endothelial cell [6, 7].
Similarly, the endothelial cells of small lymphatic capillary vessels are distinct in function and gene expression from the lymphatic endothelial cells (LEC) that line the major collecting lymphatic vessels [8]. Interestingly, Baluk et al. recently described the presence of unique cell-cell junctions in lymphatic vessels [9]. They found lymphatic capillaries had discontinuous 'button-like' junctions that would allow flaps of the vessel to open and allow fluid entry. In contrast, collecting lymphatics had continuous 'zipper' junctions, yet in both vessel types the junctions appeared to have the same molecular components. How this organisation is achieved is unknown, but it presumably stems from the functional differences of the lymphatic vessel subtypes.
Florence Sabin's pioneering work of the early 20th century mapped the development of the lymphatic vasculature by injecting blue dye into pig embryos, allowing the vessels to be visualized [10, 11]. This foundation led to recent discoveries showing that early in embryonic development, lymphatic progenitor cells migrate away from the cardinal vein [12]. The process of developmental lymphangiogenesis proceeds with vessels sprouting from the lymph sacs formed from the progenitor cells. Many molecular signals are required to stimulate the correct lymphatic network development and maturation, some of which are discussed below.
In the context of human disease, both blood and lymphatic vessels play important roles. For example, in cancer, tumor progression relies on the angiogenic switch, or the induction of new blood vessel growth [13, 14] for the supply of oxygen required for the tumor to grow. Blood vessels also provide a route for tumor dissemination to distant sites, via invasion of the bloodstream and homing to organs such as the brain, lungs, liver and bone [15]. Tumor angiogenesis (the growth of new blood vessels in a tumor) is therefore a valid target for cancer therapeutics. Recent work has shown that the lymphatic network also plays a central role in the metastasis of cancer, allowing spread to draining lymph nodes [16–18]. Clinically, many carcinomas are commonly seen to metastasize initially via the lymphatic vasculature to the lymph nodes [15], with the lymphatic vessels providing a key initial entry point for metastatic cancer cells. Numerous studies have shown a significant correlation between levels of the lymphangiogenic vascular endothelial growth factor C (VEGF-C), lymphatic vessel invasion, lymph node metastasis and/or overall survival (reviewed in [3, 15, 19]). Targeting the induction of tumor lymphangiogenesis (the generation of new lymphatic vessels within a tumor), and the signaling that drives functional changes in both new and existing lymphatic vessels (Figure 1), may help to prevent a route for tumor metastasis.
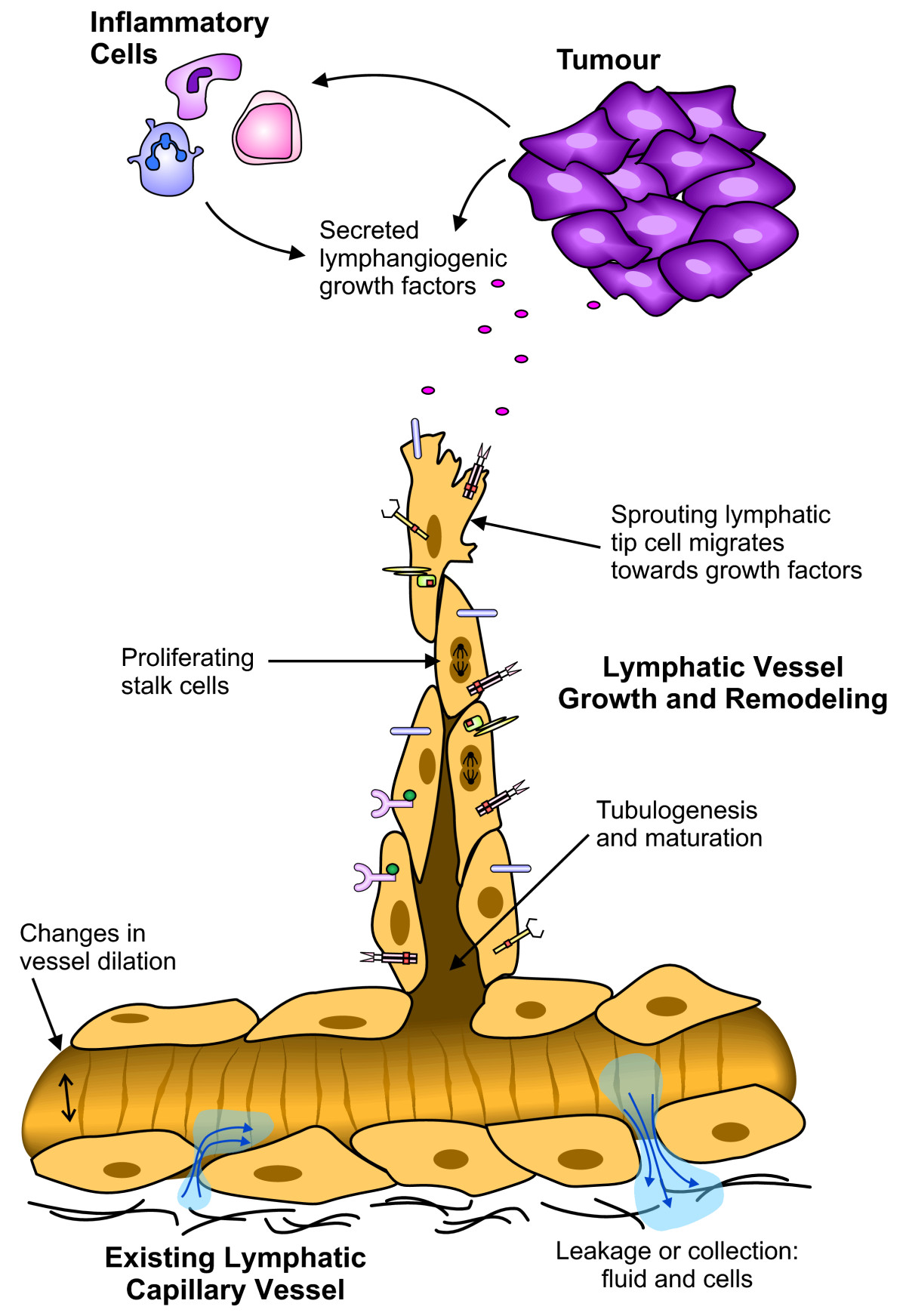
Figure 1
Figure 1 caption
Different functions of LECs in active lymphatic vessels. This schematic outlines some of the cellular processes that occur in lymphatic vessels under pathological conditions such as cancer. In this diagram a tumor (and/or infiltrating immune cells) secretes factors that induce changes in the lymphatic vasculature. Growth factors binding to the different receptors expressed on the surface of the LECs may induce sprouting of new lymphatic vessels from existing lymphatic capillaries. The leading 'tip cell' detects a gradient of growth factors by means of cell surface receptors, and migrates towards the tumor. Behind the tip cell are the stalk cells, responding to proliferation stimuli. The formation of a lumen and maturation of the vessel is required to create a functional vessel. Other aspects of the vessel such as vessel dilation and vessel permeability to fluid and cells may also be altered. These characteristics may be exaggerated in the context of a tumor, to create the abnormal vessels often associated with cancer and enhance the ease with which lymphogenous metastasis occurs. Many of these responses are induced by signaling pathways involving tyrosine kinases.
In addition to cancer, there are a range of pathological conditions associated with defective or abnormal lymphatic vessels. Lymphedema results from inadequate drainage of fluid from a limb, and can be primary or acquired. Primary lymphedema is rare, but patients are often found to harbour point mutations in key lymphatic genes such as vascular endothelial growth factor receptor 3 (VEGFR-3). Acquired lymphedema can be caused by damage or trauma to the lymphatic vessels (eg sentinel lymph node biopsy), or infection with the parasitic worms that cause filariasis (elephantiasis). Recent work by Tammela et al. [20] demonstrated that by stimulating the VEGFR-3 tyrosine kinase by treatment with the lymphangiogenic vascular endothelial growth factors C or D (VEGF-C or VEGF-D) it is possible to regenerate functional collecting lymphatic vessels in mice following lymph node dissection.
Lymphangioma or lymphangiectasia can result from a build up of fluid, causing an excessive dilation/distension of the lymphatic vessels that is not resolved. Patients (often children) may present with a group of skin lesions that discharge milky fluid, or cystic masses of the head, neck or genitals. Current treatments rely on compression bandages or surgery, although more recently sclerosing agents have been used with some success to induce fibrous obliteration of the vessel [21].
Therefore, understanding the biology of the lymphatics and lymphatic endothelium may provide new options for the treatment of diseases involving the lymphatics, such as cancer, lymphangioma, lymphedema and wound healing.
Tyrosine kinases in vascular biology
Current strategies for targeting tyrosine kinases
Therapeutic targeting of PTKs has been approached from a number of angles, with varying success. Humanized monoclonal antibodies (mAb) raised against the extracellular domains of an RTK have been used. The first FDA approved PTK inhibitor was trastuzumab, a mAb directed to the HER2/neu RTK [63, 64] for use against metastatic breast cancer. Since then, several others have made their way into the clinic; bevacizumab [65, 66], and cetuximab [67, 68] being the most significant examples. Monoclonal antibody inhibitors of RTKs act via prevention of receptor dimerization and ligand binding, and in some cases may cause receptor internalization and immune cell recruitment [64]. Antibodies generally allow much more specific blocking and thus have the advantage of specificity that small molecule inhibitors tend to lack. Inhibitory antibodies are however, only effective against cell surface receptors, and not against non-receptor tyrosine kinases.
Recent developments in medicinal chemistry and crystallography have led to the possibility of tailor-made small molecule inhibitors that are designed to fit perfectly into the active site of the kinase. These small molecules are able to enter the cell and it is therefore possible to target them to either the intracellular kinase domain of RTKs or the cytoplasmic tyrosine kinases. However one of the caveats of small molecule PTK inhibitors is that kinase domains are highly similar across the families, making selective inhibition difficult. This does mean that multiple pathways may be blocked simultaneously, which may have therapeutic benefit in some cases [27, 69]. The disadvantage of a less selective small molecule PTK inhibitor is greater toxicity and risk of adverse effects. Some PTK inhibitors are well tolerated, however reported effects are anemia, rash, diarrhea, nausea, fatigue, weight loss and hypertension [70, 71].
The prototype small molecule PTK inhibitor is imatinib; targeted to the chimeric protein that occurs in 95% of chronic myeloid leukemia patients as a result of the t(9;22)(q34;q11) translocation [72]. This fusion of the
Available strategies for anti-lymphangiogenesis therapy via PTK family
First proposed by Folkman in 1971 [2], anti-angiogenic therapy has now become accepted for cancer treatment [78]. Current strategies for targeting the blood vasculature are focused on inhibition of VEGF and/or blockade of the VEGFRs which activate the downstream pathways [71, 79]. Bevacizumab, also known as Avastin (Genentech), is a monoclonal anti-VEGF antibody that has been approved in combination with chemotherapies for colorectal cancer, non-squamous non-small cell lung cancer, metastatic renal cell carcinoma and metastatic HER2-negative breast cancer [65, 66]. Despite this, there is a risk of side effects such as gastrointestinal perforation, bleeding and impaired wound healing. Bevacizumab's exact mechanism of action is somewhat unclear, and while it may have some anti-angiogenic properties, the key may actually lie in the stabilization of tumor vessels. By normalizing the tumor vessels, the blood flow is increased and interstitial pressure is reduced allowing conventional chemotherapy better access to the tumor.
Other approaches have used soluble forms of VEGFR to create the 'VEGF-trap' (Regeneron), a recombinant chimeric decoy receptor which is in clinical trials [80, 81]. Similarly, ImClone has developed inhibitory antibodies for VEGFR-1 [82] and VEGFR-2 [83–86], both of which are in clinical trials. A human neutralizing anti-VEGFR-3 antibody has also been generated [87]; in mouse experiments an equivalent antibody to mouse VEGFR-3 was shown to completely block tumor lymphangiogenesis with no effect on preexisting vessels [88] (Table 1). Soluble VEGFR-3 and antibodies targeted to VEGF-C and VEGF-D are in commercial development. Recently, several groups have had success creating peptidomimetics in a form that are resistant to degradation [89, 90]. One of these is targeted to VEGFR-1 and NRP1, and appears effective at blocking angiogenesis in mouse models of retinopathy and cancer [90].
In contrast there are a large number of small molecule inhibitors available that inhibit VEGFR signaling [71]. However many of them also inhibit the activity of other related RTKs such as platelet derived growth factor receptors (PDGFRs), c-KIT and colony stimulating factor 1 receptor (CSF1R) due to similarity in the kinase site, and it is not uncommon to show activity against a wider range of kinases. The VEGF receptor inhibitors that have been FDA approved as chemotherapeutics are sorafenib (Bayer) [91, 92], sunitinib (Pfizer) [93–95] and pazopanib (GlaxoSmithKline) [96]. One of the commonly seen issues associated with all anti-VEGF treatments is resistance, as alternative proangiogenic pathways are switched on. Small molecule inhibitors that target multiple pathways (e.g. VEGFRs, FGFRs and PDGFRs) simultaneously may avoid this problem, but also increase the risk of associated side-effects. Sorafenib was originally designed to inhibit B-Raf, and was found to be effective in renal and hepatocellular cancers. However, this is now attributed not to the inhibition of B-Raf, but to its activity against VEGFR-2 and PDGFRβ [69]. This leads to blockade of angiogenesis through VEGFR-2, and PDGFRβ inhibition prevents the recruitment of pericytes for vessel stabilization. Recently Murphy et al. [97] reported a second generation 'Type II' inhibitor, designed to be highly selective for PDGFRβ and B-Raf. Oral administration of this compound was able to suppress growth of orthotopic kidney and pancreatic tumors in mice, with significant anti-angiogenic effects.
Eph-Ephrin signaling is a promising anti-angiogenic/anti-lymphangiogenic target. A number of small molecule inhibitors are available [59], including EXEL-7647. EXEL-7647 is currently in clinical trials, and inhibits epidermal growth factor receptor (EGFR), ErbB2, VEGFRs and EphB4 [98, 99]. Other inhibitors in the form of peptidomimetics, inhibitory monoclonal antibodies, and soluble receptors are being tested [59]. It also remains to investigate in more detail the contribution of other Eph receptors to vascular biology; EphA2 signaling has been shown to contribute to tumor angiogenesis, while the ligand ephrinA1 can be upregulated by VEGF [100]. This complex field of Eph signaling, if well understood, could give rise to a range of useful therapeutics.
The nine Src family kinases are cytoplasmic PTKs closely associated with the cell membrane and both RTKs and non-PTK receptors (Figure 2). Src family kinases mediate signal transduction pathways relating to many critical functions of a cell; proliferation, apoptosis, cell adhesion and migration [25]. A number of small molecule inhibitors are available, and several are in clinical trials [25]. Inhibitors of Src family kinases may be useful both to reduce the expression of growth factors in tumor cells [101], as well as having direct effects on the endothelium. Src is known to interact with VEGF receptors, and a selective Src inhibitor significantly reduced human umbilical vein endothelial cell (HUVEC) proliferation and migration
Currently there are no PTK inhibitors specifically targeting the lymphatics. Even VEGFR-3, which was thought to be specific to LECs, has now been shown to be expressed at the leading edge of sprouting blood vasculature [105]. Therefore this remains an attractive target for dual inhibition of blood and lymphatic growth [105]. Encouragingly, it was recently shown that inhibition of the coreceptor NRP2 specifically blocked lymphatic vessel sprouting and migration but did not affect cell proliferation [40, 106]. As many of the trials of PTK inhibitors have been focused on anti-angiogenic efficacy, it remains to be determined whether any possess significant anti-lymphangiogenic potential. Evaluation of specific inhibitors will be required to identify those that have activity in
Identifying new targets for anti-lymphatic treatment
In order to identify new targets for anti-lymphangiogenic treatments efficiently, screening strategies must be successfully employed. The recent and exciting advent of RNAi technology and high throughput screening systems have allowed researchers to investigate the functional importance of a large number of genes in
High throughput screening of chemical libraries offers the opportunity to screen thousands of compounds to identify small molecule inhibitors of a cell process of interest [126–129]. If a key kinase target is known, the assay readout can be set to indicate whether the compound is on-target [130]. Chemical library screens are commonly performed
Alternatively, once a target has been identified, rational drug design can be used to develop a compound that binds with high specificity [77]. This approach has been used to create drugs such as imatinib, but also more recently a selective inhibitor of both B-Raf and PDGFRβ [97]. Finding the balance between highly selective compounds and still inhibiting the multiple necessary pathways to see maximal effect without causing severe side-effects will require a combination of approaches. RNAi screening allows the entire genome to be screened, including the thousands of virtually unannotated genes. Similarly, chemical libraries now comprise hundreds of thousands of compounds, many of which are unknown. These platform technologies may soon provide targets and lead compounds, and eventually give rise to reagents targeting protein tyrosine kinases for anti-lymphangiogenic therapy that have clinical application.
Conclusions
The recent renaissance in lymphatic endothelial biology has led to a better understanding of the important role these vessels play in health and disease. It is now apparent that specific targeting of protein tyrosine kinases is an effective way to elicit anti-angiogenic responses in the context of cancer therapy. Similar approaches could be used to target lymphatics to prevent metastasis, while in other pathological conditions such as lymphedema, targeted therapy may be used to restore their growth and subsequent function. Some of these treatments have been developed to existing targets such as the VEGFRs and their ligands. Further testing will be required to fully determine their efficacy, but there are also potentially many novel targets not yet discovered or not currently associated with lymphatic biology.
Acknowledgements
This work was funded partly by a Program Grant from the National Health and Medical Research Council of Australia (NH&MRC). SAS and MGA are supported by Senior Research Fellowships from the NH&MRC. SAS would like to acknowledge the support of the Pfizer Australia Fellowship. SPW is supported by a National Breast Cancer Foundation Doctoral Research Scholarship. The authors thank J. Taylor for assistance in generating figures.
Authors’ original submitted files for images
Below are the links to the authors’ original submitted files for images.
Authors’ original file for figure 1
Authors’ original file for figure 2
References
- Lymphangiogenesis: Molecular mechanisms and future promise. Cell. 2010;140:460-476.
- Tumor angiogenesis: therapeutic implications. N Engl J Med. 1971;285:1182-1186.
- Lymphangiogenesis and cancer metastasis. Nat Rev Cancer. 2002;2:573-583.
- VEGF receptor signalling - in control of vascular function. Nat Rev Mol Cell Biol. 2006;7:359-371.
- Molecular regulation of angiogenesis and lymphangiogenesis. Nat Rev Mol Cell Biol. 2007;8:464-478.
- Isolation and characterization of dermal lymphatic and blood endothelial cells reveal stable and functionally specialized cell lineages. J Exp Med. 2001;194:797-808.
- Molecular characterization of lymphatic endothelial cells. Proc Natl Acad Sci USA. 2002;99:16069-16074.
- Lymphatic vessels in cancer metastasis: bridging the gaps. Carcinogenesis. 2006;27:1729-1738.
- Functionally specialized junctions between endothelial cells of lymphatic vessels. J Exp Med. 2007;204:2349-2362.
- On the origin of the lymphatic system from the veins and the development of the lymph hearts and thoracic duct in the pig. American Journal of Anatomy. 1902;1:367-389.
- On the development of the superficial lymphatics in the skin of the pig. American Journal of Anatomy. 1904;3:183-195.
- Lymphatic vasculature development. Nat Rev Immunol. 2004;4:35-45.
- The angiogenic switch in carcinogenesis. Semin Cancer Biol. 2009;19:329-337.
- Role of angiogenesis in human tumor dormancy: animal models of the angiogenic switch. Cell Cycle. 2006;5:1779-1787.
- Clinical patterns of metastasis. Cancer Metastasis Rev. 2006;25:221-232.
- Vascular endothelial growth factor-C-mediated lymphangiogenesis promotes tumour metastasis. EMBO J. 2001;20:672-682.
- Induction of tumor lymphangiogenesis by VEGF-C promotes breast cancer metastasis. Nat Med. 2001;7:192-198.
- VEGF-D promotes the metastatic spread of tumor cells via the lymphatics. Nat Med. 2001;7:186-191.
- Lymphangiogenesis and lymphatic metastasis in breast cancer. Pathophysiology. 2009.
- Therapeutic differentiation and maturation of lymphatic vessels after lymph node dissection and transplantation. Nat Med. 2007;13:1458-1466.
- Treatment of lymphangioma in children: our experience of 128 cases. J Pediatr Surg. 2007;42:386-389.
- The protein tyrosine kinase family of the human genome. Oncogene. 2000;19:5548-5557.
- The protein kinase complement of the human genome. Science. 2002;298:1912-1934.
- A renaissance for SRC. Nat Rev Cancer. 2004;4:470-480.
- Src kinases as therapeutic targets for cancer. Nat Rev Clin Oncol. 2009;6:587-595.
- Presenilin-dependent ErbB4 nuclear signaling regulates the timing of astrogenesis in the developing brain. Cell. 2006;127:185-197.
- Targeting the cancer kinome through polypharmacology. Nat Rev Cancer. 2010;10:130-137.
- Targeting tumor stroma. Curr Cancer Drug Targets. 2008;8:446-.
- VEGF-mediated signal transduction in lymphatic endothelial cells. Pathophysiology. 2009.
- Identification of the KDR tyrosine kinase as a receptor for vascular endothelial cell growth factor. Biochem Biophys Res Commun. 1992;187:1579-1586.
- The fms-like tyrosine kinase, a receptor for vascular endothelial growth factor. Science. 1992;255:989-991.
- Vascular endothelial growth factor D (VEGF-D) is a ligand for the tyrosine kinases VEGF receptor 2 (Flk1) and VEGF receptor 3 (Flt4). Proc Natl Acad Sci USA. 1998;95:548-553.
- A novel vascular endothelial growth factor, VEGF-C, is a ligand for the Flt4 (VEGFR-3) and KDR (VEGFR-2) receptor tyrosine kinases. Embo J. 1996;15:290-298.
- Isolated lymphatic endothelial cells transduce growth, survival and migratory signals via the VEGF-C/D receptor VEGFR-3. EMBO J. 2001;20:4762-4773.
- Vascular endothelial growth factor-C-mediated lymphangiogenesis promotes tumour metastasis. EMBO J. 2001;20:672-682.
- Deletion of vascular endothelial growth factor C (VEGF-C) and VEGF-D is not equivalent to VEGF receptor 3 deletion in mouse embryos. Mol Cell Biol. 2008;28:4843-4850.
- Ligand-induced vascular endothelial growth factor receptor-3 (VEGFR-3) heterodimerization with VEGFR-2 in primary lymphatic endothelial cells regulates tyrosine phosphorylation sites. J Biol Chem. 2003;278:40973-40979.
- VEGF receptor 2/-3 heterodimers detected in situ by proximity ligation on angiogenic sprouts. EMBO J. 2010;29:1377-1388.
- Vascular endothelial growth factor receptor-2 and neuropilin-1 form a receptor complex that is responsible for the differential signaling potency of VEGF(165) and VEGF(121). J Biol Chem. 2001;276:25520-25531.
- Neuropilin-2 mediates VEGF-C-induced lymphatic sprouting together with VEGFR3. J Cell Biol. 2010;188:115-130.
- Neuropilin-2 interacts with VEGFR-2 and VEGFR-3 and promotes human endothelial cell survival and migration. Blood. 2006;108:1243-1250.
- Functional interaction of VEGF-C and VEGF-D with neuropilin receptors. FASEB J. 2006;20:1462-1472.
- Neuropilin-1 modulates p53/caspases axis to promote endothelial cell survival. PLoS One. 2007;2:e1161-.
- Tumor lymphangiogenesis and metastatic spread-new players begin to emerge. Int J Cancer. 2006;119:1755-1760.
- Hepatocyte growth factor is a lymphangiogenic factor with an indirect mechanism of action. Blood. 2006;107:3531-3536.
- Hepatocyte growth factor promotes lymphatic vessel formation and function. EMBO J. 2005;24:2885-2895.
- Prox1 promotes lineage-specific expression of fibroblast growth factor (FGF) receptor-3 in lymphatic endothelium: a role for FGF signaling in lymphangiogenesis. Mol Biol Cell. 2006;17:576-584.
- Insulin-like growth factors 1 and 2 induce lymphangiogenesis in vivo. Proc Natl Acad Sci USA. 2005;102:15593-15598.
- Role of Angiopoietins and Tie receptor tyrosine kinases in angiogenesis and lymphangiogenesis. Cell Tissue Res. 2003;314:61-68.
- Differential response of lymphatic, venous and arterial endothelial cells to angiopoietin-1 and angiopoietin-2. BMC Cell Biol. 2007;8:10-.
- Dominant-negative and targeted null mutations in the endothelial receptor tyrosine kinase, tek, reveal a critical role in vasculogenesis of the embryo. Genes Dev. 1994;8:1897-1909.
- Distinct roles of the receptor tyrosine kinases Tie-1 and Tie-2 in blood vessel formation. Nature. 1995;376:70-74.
- Requisite role of angiopoietin-1, a ligand for the TIE2 receptor, during embryonic angiogenesis. Cell. 1996;87:1171-1180.
- The receptor tyrosine kinase TIE is required for integrity and survival of vascular endothelial cells. EMBO J. 1995;14:5884-5891.
- Loss of endothelial Tie1 receptor impairs lymphatic vessel development-brief report. Arterioscler Thromb Vasc Biol. 2010;30:207-209.
- Failure of blood-island formation and vasculogenesis in Flk-1-deficient mice. Nature. 1995;376:62-66.
- Angiopoietin-2 is required for postnatal angiogenesis and lymphatic patterning, and only the latter role is rescued by Angiopoietin-1. Dev Cell. 2002;3:411-423.
- Regulation of angiogenesis by Eph-ephrin interactions. Trends Cardiovasc Med. 2007;17:145-151.
- Eph receptors and ephrins in cancer: bidirectional signalling and beyond. Nat Rev Cancer. 2010;10:165-180.
- PDZ interaction site in ephrinB2 is required for the remodeling of lymphatic vasculature. Genes Dev. 2005;19:397-410.
- Artery and vein size is balanced by Notch and ephrin B2/EphB4 during angiogenesis. Development. 2008;135:3755-3764.
- Prox1 induces lymphatic endothelial differentiation via integrin alpha9 and other signaling cascades. Mol Biol Cell. 2007;18:1421-1429.
- Trastuzumab, a recombinant DNA-derived humanized monoclonal antibody, a novel agent for the treatment of metastatic breast cancer. Clin Ther. 1999;21:309-318.
- Trastuzumab--mechanism of action and use in clinical practice. N Engl J Med. 2007;357:39-51.
- Humanization of an anti-vascular endothelial growth factor monoclonal antibody for the therapy of solid tumors and other disorders. Cancer Res. 1997;57:4593-4599.
- A randomized trial of bevacizumab, an anti-vascular endothelial growth factor antibody, for metastatic renal cancer. N Engl J Med. 2003;349:427-434.
- Biological efficacy of a chimeric antibody to the epidermal growth factor receptor in a human tumor xenograft model. Clin Cancer Res. 1995;1:1311-1318.
- IMC-C225, an anti-epidermal growth factor receptor monoclonal antibody for treatment of head and neck cancer. Semin Oncol. 2002;29:18-30.
- Benefits of targeting both pericytes and endothelial cells in the tumor vasculature with kinase inhibitors. J Clin Invest. 2003;111:1287-1295.
- Emerging roles of targeted small molecule protein-tyrosine kinase inhibitors in cancer therapy. Oncol Res. 2004;14:175-225.
- An overview of small-molecule inhibitors of VEGFR signaling. Nat Rev Clin Oncol. 2009;6:569-579.
- Unique fusion of bcr and c-abl genes in Philadelphia chromosome positive acute lymphoblastic leukemia. Cell. 1987;51:33-40.
- An alteration of the human c-abl protein in K562 leukemia cells unmasks associated tyrosine kinase activity. Cell. 1984;37:1035-1042.
- Inhibition of the Abl protein-tyrosine kinase in vitro and in vivo by a 2-phenylaminopyrimidine derivative. Cancer Res. 1996;56:100-104.
- Effects of a selective inhibitor of the Abl tyrosine kinase on the growth of Bcr-Abl positive cells. Nat Med. 1996;2:561-566.
- Structural mechanism for STI-571 inhibition of abelson tyrosine kinase. Science. 2000;289:1938-1942.
- Rational design of inhibitors that bind to inactive kinase conformations. Nat Chem Biol. 2006;2:358-364.
- VEGF-targeted therapy: mechanisms of anti-tumour activity. Nat Rev Cancer. 2008;8:579-591.
- Drug insight: VEGF as a therapeutic target for breast cancer. Nat Clin Pract Oncol. 2007;4:181-189.
- Use of soluble recombinant decoy receptor vascular endothelial growth factor trap (VEGF Trap) to inhibit vascular endothelial growth factor activity. Clin Colorectal Cancer. 2004;4(Suppl 2):S81-85.
- Phase I study of intravenous vascular endothelial growth factor trap, aflibercept, in patients with advanced solid tumors. J Clin Oncol. 2010;28:207-214.
- Anti-vascular endothelial growth factor receptor-1 antagonist antibody as a therapeutic agent for cancer. Clin Cancer Res. 2006;12:6573-6584.
- Monoclonal antibodies targeting vascular endothelial growth factor: current status and future challenges in cancer therapy. BioDrugs. 2009;23:289-304.
- Ramucirumab, a fully human mAb to the transmembrane signaling tyrosine kinase VEGFR-2 for the potential treatment of cancer. Curr Opin Investig Drugs. 2009;10:597-605.
- TRIO-012: a multicenter, multinational, randomized, double-blind phase III study of IMC-1121B plus docetaxel versus placebo plus docetaxel in previously untreated patients with HER2-negative, unresectable, locally recurrent or metastatic breast cancer. Clin Breast Cancer. 2009;9:258-261.
- Phase I pharmacologic and biologic study of ramucirumab (IMC-1121B), a fully human immunoglobulin G1 monoclonal antibody targeting the vascular endothelial growth factor receptor-2. J Clin Oncol. 2010;28:780-787.
- Involvement of the VEGF receptor 3 in tubular morphogenesis demonstrated with a human anti-human VEGFR-3 monoclonal antibody that antagonizes receptor activation by VEGF-C. J Cell Sci. 2004;117:2745-2756.
- Complete and specific inhibition of adult lymphatic regeneration by a novel VEGFR-3 neutralizing antibody. J Natl Cancer Inst. 2005;97:14-21.
- From combinatorial peptide selection to drug prototype (II): Targeting the epidermal growth factor receptor pathway. Proc Natl Acad Sci USA. 2010;107:5118-5123.
- From combinatorial peptide selection to drug prototype (I): Targeting the vascular endothelial growth factor receptor pathway. Proc Natl Acad Sci USA. 2010;107:5112-5117.
- Safety, pharmacokinetics, and preliminary antitumor activity of sorafenib: a review of four phase I trials in patients with advanced refractory solid tumors. Oncologist. 2007;12:426-437.
- Phase I clinical and pharmacokinetic study of the Novel Raf kinase and vascular endothelial growth factor receptor inhibitor BAY 43-9006 in patients with advanced refractory solid tumors. J Clin Oncol. 2005;23:965-972.
- In vivo antitumor activity of SU11248, a novel tyrosine kinase inhibitor targeting vascular endothelial growth factor and platelet-derived growth factor receptors: determination of a pharmacokinetic/pharmacodynamic relationship. ClinCancer Res. 2003;9:327-337.
- Activity of SU11248, a multitargeted inhibitor of vascular endothelial growth factor receptor and platelet-derived growth factor receptor, in patients with metastatic renal cell carcinoma. J Clin Oncol. 2006;24:16-24.
- Safety, pharmacokinetic, and antitumor activity of SU11248, a novel oral multitarget tyrosine kinase inhibitor, in patients with cancer. J Clin Oncol. 2006;24:25-35.
- Pharmacokinetic-pharmacodynamic correlation from mouse to human with pazopanib, a multikinase angiogenesis inhibitor with potent antitumor and antiangiogenic activity. Mol Cancer Ther. 2007;6:2012-2021.
- Disruption of angiogenesis and tumor growth with an orally active drug that stabilizes the inactive state of PDGFRb/B-RAF. Proc Natl Acad Sci USA. 2010;107:4299-4304.
- Inhibition of the T790 M gatekeeper mutant of the epidermal growth factor receptor by EXEL-7647. Clin Cancer Res. 2007;13:3713-3723.
- Combined inhibition of the VEGFR and EGFR signaling pathways in the treatment of NSCLC. Oncologist. 2009;14:399-411.
- Blockade of EphA receptor tyrosine kinase activation inhibits vascular endothelial cell growth factor-induced angiogenesis. Mol Cancer Res. 2002;1:2-11.
- AP23846, a novel and highly potent Src family kinase inhibitor, reduces vascular endothelial growth factor and interleukin-8 expression in human solid tumor cell lines and abrogates downstream angiogenic processes. Mol Cancer Ther. 2005;4:1900-1911.
- A novel Src kinase inhibitor, M475271, inhibits VEGF-induced human umbilical vein endothelial cell proliferation and migration. J Pharmacol Sci. 2005;98:130-141.
- Src tyrosine kinase inhibition suppresses lymphangiogenesis in vitro and in vivo. Curr Cancer Drug Targets. 2010;10:546-553.
- Effect of Src kinase inhibition on metastasis and tumor angiogenesis in human pancreatic cancer. Angiogenesis. 2007;10:167-182.
- Blocking VEGFR-3 suppresses angiogenic sprouting and vascular network formation. Nature. 2008;454:656-660.
- Blocking neuropilin-2 function inhibits tumor cell metastasis. Cancer Cell. 2008;13:331-342.
- The art and design of genetic screens: RNA interference. Nat Rev Genet. 2008;9:554-566.
- Systematic genome-wide screens of gene function. Nat Rev Genet. 2004;5:11-22.
- High-throughput RNAi screening in cultured cells: a user's guide. Nat Rev Genet. 2006;7:373-384.
- Genome-wide functional analysis of human cell-cycle regulators. Proc Natl Acad Sci USA. 2006;103:14819-14824.
- Genome-scale RNAi profiling of cell division in human tissue culture cells. Nat Cell Biol. 2007;9:1401-1412.
- Identification of modulators of TRAIL-induced apoptosis via RNAi-based phenotypic screening. Mol Cell. 2003;12:627-637.
- Genome-wide analysis of human kinases in clathrin-and caveolae/raft-mediated endocytosis. Nature. 2005;436:78-86.
- A small interfering RNA screen for modulators of tumor cell motility identifies MAP4K4 as a promigratory kinase. Proc Natl Acad Sci USA. 2006;103:3775-3780.
- Modular control of endothelial sheet migration. Genes Dev. 2008;22:3268-3281.
- Identification of genes that regulate epithelial cell migration using an siRNA screening approach. Nat Cell Biol. 2008;10:1027-1038.
- A functional genomic analysis of cell morphology using RNA interference. J Biol. 2003;2:27-.
- Identification of neural outgrowth genes using genome-wide RNAi. PLoS Genet. 2008;4:e1000111-.
- Loss-of-function genetic screens as a tool to improve the diagnosis and treatment of cancer. Oncogene. 2009;28:4409-4420.
- High-throughput approaches to dissecting MAPK signaling pathways. Methods. 2006;40:262-271.
- Identification of Hedgehog pathway components by RNAi in Drosophila cultured cells. Science. 2003;299:2039-2045.
- A large-scale RNAi screen in human cells identifies new components of the p53 pathway. Nature. 2004;428:431-437.
- A functional RNAi screen for regulators of receptor tyrosine kinase and ERK signalling. Nature. 2006;444:230-234.
- Identification of JAK/STAT signalling components by genome-wide RNA interference. Nature. 2005;436:871-875.
- Evidence of RNAi in humans from systemically administered siRNA via targeted nanoparticles. Nature. 2010;464:1067-1070.
- Identification of selective inhibitors of cancer stem cells by high-throughput screening. Cell. 2009;138:645-659.
- Screening for cell migration inhibitors via automated microscopy reveals a Rho-kinase inhibitor. Chem Biol. 2005;12:385-395.
- Phenotypic screening of small molecule libraries by high throughput cell imaging. Comb Chem High Throughput Screen. 2003;6:279-286.
- An efficient rapid system for profiling the cellular activities of molecular libraries. Proc Natl Acad Sci USA. 2006;103:3153-3158.
- SU5416 is a potent and selective inhibitor of the vascular endothelial growth factor receptor (Flk-1/KDR) that inhibits tyrosine kinase catalysis, tumor vascularization, and growth of multiple tumor types. Cancer Research. 1999;59:99-106.
- An in vivo chemical library screen in Xenopus tadpoles reveals novel pathways involved in angiogenesis and lymphangiogenesis. Blood. 2009;114:1110-1122.
- Alternatively spliced vascular endothelial growth factor receptor-2 is an essential endogenous inhibitor of lymphatic vessel growth. Nat Med. 2009;15:1023-1030.
- Differential effects of the vascular endothelial growth factor receptor inhibitor PTK787/ZK222584 on tumor angiogenesis and tumor lymphangiogenesis. Mol Cancer Ther. 2009;8:55-63.
- Inhibition of lymphangiogenesis with resulting lymphedema in transgenic mice expressing soluble VEGF receptor-3. Nat Med. 2001;7:199-205.
- Inflammatory corneal (lymph)angiogenesis is blocked by VEGFR-tyrosine kinase inhibitor ZK 261991, resulting in improved graft survival after corneal transplantation. Invest Ophthalmol Vis Sci. 2008;49:1836-1842.
- The tyrosine kinase inhibitor cediranib blocks ligand-induced vascular endothelial growth factor receptor-3 activity and lymphangiogenesis. Cancer Res. 2008;68:4754-4762.
- Contrasting actions of selective inhibitors of angiopoietin-1 and angiopoietin-2 on the normalization of tumor blood vessels. Am J Pathol. 2009;175:2159-2170.
- PDGF-BB induces intratumoral lymphangiogenesis and promotes lymphatic metastasis. Cancer Cell. 2004;6:333-345.
- Anticancer strategies involving the vasculature. Nat Rev Clin Oncol. 2009;6:395-404.