Vaccines targeting the neovasculature of tumors
Vascular Cell. 2011;
Received: 22 November 2010 | Accepted: 8 March 2011 | Published: 8 March 2011
Vascular Cell ISSN: 2045-824X
Abstract
Angiogenesis has a critical role in physiologic and disease processes. For the growth of tumors, angiogenesis must occur to carry sufficient nutrients to the tumor. In addition to growth, development of new blood vessels is necessary for invasion and metastases of the tumor. A number of strategies have been developed to inhibit tumor angiogenesis and further understanding of the interplay between tumors and angiogenesis should allow new approaches and advances in angiogenic therapy. One such promising angiogenic approach is to target and inhibit angiogenesis with vaccines. This review will discuss recent advances and future prospects in vaccines targeting aberrant angiogenesis of tumors. The strategies utilized by investigators have included whole endothelial cell vaccines as well as vaccines with defined targets on endothelial cells and pericytes of the developing tumor endothelium. To date, several promising anti-angiogenic vaccine strategies have demonstrated marked inhibition of tumor growth in pre-clinical trials with some showing no observed interference with physiologic angiogenic processes such as wound healing and fertility.
Introduction
Cancer mortality is related to the spread of neoplastic cells to distant loci where the cells, supported by existing blood vessels and angiogenesis, proliferate and give rise to secondary tumors. Tumor angiogenesis is up-regulated by a number of conditions including hypoxia, hypoglycemia, mechanical disruption, and genetic and inflammatory alterations [1] that lead to activation of growth factors and pro-angiogenic genes [2, 3]. The stringent regulation of angiogenesis in normal tissues is often lacking in tumor angiogenesis, resulting in immature and leaky tumor vessels. Furthermore, compared to the tissue-vessel distribution in normal tissue, there is an uneven distribution of vessels within tumors, leading to tumor hypoxia and inefficient transport of chemotherapeutic drugs. In contrast to normal endothelial cells, in which the vast majority are quiescent, tumor endothelial cells actively proliferate, driven by hypoxia and increased levels of angiogenic factors and their cognate receptors. These differences between quiescent and angiogenic endothelial cells resulted in the first clinical anti-angiogenesis trial on human cancer two decades ago. There are now several anti-angiogenic therapies that have received FDA approval including sunitinib, sorafenib, and bevacizumab; and with more than 40 anti-angiogenic drugs in clinical trials [4], further advances are anticipated [5–11].
Differences among tumor endothelial cells and non-malignant endothelial cells may not only be quantitative but in some instances may also be qualitative. With serial analysis of gene expression, investigators compared gene expression from endothelial cells isolated from normal or malignant tissue, and found that several transcripts (e.g., CD276) were specifically elevated in the tumor endothelium [12, 13]. Although most receptors/proteins that are increased in the tumor endothelium are also up-regulated in physiologic angiogenic processes, CD276 is not increased in the vessels of wounds or the corpus luteum [13]. Nevertheless, CD276 is not completely specific for the tumor endothelium because its expression may be induced by cytokines on the cell surfaces of B cells, T cells, and dendritic cells. There are also many proteins/receptors in tumor endothelial cells that are overexpressed (such as VEGFR2 and survivin) compared to expression in quiescent endothelial cells. Proteins differentially expressed on tumor endothelial cells or the supporting matrix are attractive targets for vaccine strategies, with the goal of breaking tolerance to self-antigens.
Targeting the tumor vasculature with vaccines as well as with other immunotherapies may have several potential advantages over targeting tumor cells. First, tumor endothelial cells are more accessible to the immune system than are tumor cells at a distance from the vessels. Second, endothelial cells of the tumor are usually more stable genetically than tumor cells, thereby reducing the risk of resistance developing to immunotherapies [14, 15]. Chromosomal abnormalities, however, have been identified in endothelial cells of solid tumors [16, 17], and in glioblastomas, the tumor cells and its endothelium are derived from common cancer stem-like cells [18, 19]. Third, down-regulation of MHC I in tumor cells occurs less frequently in tumor endothelial cells, thereby leading to a more potent CD8+-mediated response. Fourth, since inhibition of a single endothelial cell can inhibit up to 100 tumor cells [20, 21], immunotherapies directed toward tumor endothelial cells have the potential of an amplifying inhibitory effect.
As a result of these putative advantages and differentially expressed proteins in the tumor endothelium, a number of immunotherapeutic strategies have targeted angiogenesis, including monoclonal antibodies, vaccinations, and adjuvant co-stimulatory therapies [1]. The most successful of these approaches, thus far, has been passive immunotherapy by utilizing monoclonal antibodies. In 2004, the monoclonal antibody bevacizumab which targets angiogenesis through VEGF received approval for treatment of colorectal cancer [22]. Bevacizumab has also shown efficacy against other cancers including lung, renal, and breast cancers [23, 24]. It is likely that the success and ability of bevacizumab to selectively target tumor endothelial cells has provided impetus to development of other forms of angiogenic immunotherapies. Several promising preclinical studies of tumor endothelial vaccines have led to clinical trials that are primarily in phase I. In the burgeoning field of tumor immunotherapies, we will focus on tumor vaccines that have a major anti-angiogenic component.
Delivery Systems of Tumor Endothelial Vaccines
As this review will highlight, there are many promising tumor endothelial vaccines with demonstrated efficacy in various animal models. These vaccines have been delivered by different approaches/vectors, including direct inoculation of peptides or "naked DNA", gene gun with gold particles, intradermal electroporation, tumor or dendritic cell-based vectors, and attenuated live bacteria vectors. The particular delivery system for anti-angiogenic vaccine therapy is selected at least in part based on whether immunizations are comprised of peptides/proteins, DNA, or RNA. For peptide delivery systems, the peptide can be inoculated directly into the animal model along with an adjuvant, or dendritic cells can be pulsed with the peptides before their inoculation. For gene therapy vaccine approaches, recombinant DNA may be delivered alone ("naked DNA"), by non-viral and viral carriers, or by eukaryotic and prokaryotic cells. Although delivery systems for vaccines targeting tumor endothelial cells generally mirror those targeting tumor cells [25–29], there are exceptions such as the infrequent use of viruses with tumor-endothelial vaccines. Nevertheless, we see no contraindication to using modified herpes simplex or vaccinia viruses to augment the immune response of endothelial vaccines as in tumor cell vaccines.
To date, plasmids encoding angiogenic self-antigens are the most common forms of nucleic acid to demonstrate an anti-angiogenic effect in mouse models. Moreover, bacteria have been the most frequently used delivery system for plasmid-based vaccines (see reviews of [30, 31]). Of the 32 vaccines with specific targets covered in this review, bacteria were the primary delivery vector in 11 studies, whereas direct inoculation of "naked" plasmid DNA was the primary delivery system in 6 studies (see Table 1). Several animal studies have demonstrated that orally administered bacteria-based vectors with attenuated, nonreplicating strains of
Table 1
Different Strategies Utilized With Tumor Endothelial Vaccines
TARGET | FORM OF THE VACCINE | TUMORS | Vector/Route | MECHANISMS | Other Comments | REFERENCES |
---|---|---|---|---|---|---|
VEGFR2 | mVEGFR2-AP fusion protein | Melanoma and lung carcinoma | DC pulsed | Ab, CTL | P | Li Y et al., 2002 |
Autologous DNA vaccine- full-length mVEGFR2 | Melanoma, colon carcinoma, non-small cell lung carcinoma, hepatoma | CTL | Modest delay in wound healing | Niethammer AG et al., 2002 [ | ||
Xenogeneic DNA vaccine | Murine melanoma, carcinoma, fibrosarcoma, lyphoma | "Naked" DNA, | Ab, CTL, CD4+(Th1)-mediated | Quail VEGFR2 vaccine | Liu, J-Y et al., 2003 | |
Autologous DNA vaccine- mVEGFR2 fragment | Breast tumor-rat | CD8+ mediated Inf-γ Elispot | Encodes listerolysin-VEGFR2 fragment; No effect on wound healing or pregnancy | Seavey MM et al., 2009 [ | ||
Autologous DNA minigene | Murine breast and colon carcinomas | CTL | Encodes H-2Kd or H-2Dd restricted peptides | Luo Y et al., 2007 [ | ||
Autologous DNA minigene | Murine lung, prostate, and breast cancers | CTL | Plasmid also encodes HIV-TAT peptide | Zhou H et al., 2005 [ | ||
H-2Db -restricted Peptides | Murine colon carcinomas | SC | CTL | adjuvant (GM-CSF, CD40 Ab); | Dong Y et al., 2006 | |
HLA-A2 or-A24 restricted hVEGFR2 Peptides | Mouse melanoma and colon carcinomas | ID | CTL | HLA-24 restricted Peptide 169 (RFVPDGNRI) induced human PBMC-CTL lysis of endothelial cells | Wada S et al., 2005 [ | |
VEGFR2 Peptide 169 + gemcitabine | Pancreatic cancer | SC | CTL; | Adjuvant (IFA) | Miyazawa M. et al., 2009 [ | |
Xenogeneic DNA vaccine | Murine breast and colon carcinoma | Cationic liposomes, IV | Ab, CTL | Human VEGFR2; | Xie K et al., 2009 [ | |
Autologous DNA vaccine (VEGFR2 fused with β-defensin 2) | Murine lung and colon cancer | Cationic liposomes, IM | Ab, CTL | Antitumor and anti-angiogenic synergy between VEGFR2 and β-defensin-2; | Wang YS et al., 2007 [ | |
Autologous DNA vaccine- Extracellular Domain | Murine Lung | Ab, CTL CD4+ (Th1), C8+ mediated | Increased levels of IgG2a and 2b | Zuo SG et al., 2010 [ | ||
VEGF | Xenogeneic DNA | Fibrosarcoma, breast cancer, hepatoma | "Naked" DNA, | Ab | Xenopus VEGF has about 75% homology with humans and mice | Wei YQ et al., 2001 [ |
Autologous or xenogeneic protein | Murine and human colon caricinoma; human rhadosarcoma | IM | Ab; | h- or mVEGF conjugated to KLH | Rad FH et al., 2007 [ | |
FGFR-1/bFGF | Autologous bFGF peptide | Murine melanoma and lung carcinoma | Lipid A containing liposomes | Ab | Effective vaccine against the 44 aa segment of the heparin binding domain; No effect on wound healing or pregancy | Plum SM et al., 2000 [ |
Xenogeneic DNA | Murine fibrosarcoma, hepatoma and breast cancer | "Naked" DNA, IM | Ab | FGFR-1 from Xenopus laevis | He QM et al., 2003 [ | |
TEM8 | Autologous TEM8 with rat Her2 or human tyrosinase-related protein1 DNA vaccine | Rat Her-2 expressing breast carcinoma; | Gold-particle gene gun | No Ab or CTL response with TEM8 vaccine alone | Synergy observed | Felicetti P et al., 2007 [ |
Xenogeneic DNA vaccine | Murine melanoma | CTL; | Human TEM8 | Ruan Z et al., 2009 [ | ||
ENDOGLIN (CD105) | Xenogeneic protein | Murine lung, melanoma, colon carcinoma, fibrosarcoma | SC | Ab; | Synergy with cis-platinum; adjuvant (alum) | Tan GH et al., 2004 [ |
ANGIOMOTIN | Xenogeneic DNA vaccine, full-length | Her-2 expressing breast cancer in transgenic mice | Electroporation, | Ab | Human angio-motin and Her-2; antitumor synergy when combined with Her-2 DNA vaccine | Holmgren L et al., 2006 [ |
TIE2 | Xenogeneic protein vaccine | Murine hepatomas and melanomas | SC | Ab | Chicken Tie2 | Luo Y et al., 2006 [ |
DNA vaccine encoding HLA-restricted peptides | Gold-particle gene gun | CTL | HLA-A*0201/Kb transgenic mice; the epitope | Ramage JM et al., 2004 [ | ||
HP59/SP55 | Xenogeneic peptides | Murine lung carcinoma | Not stated | Ab | HP59 and SP55 peptide mixture | Fu C et al., 2001 [ |
HMW-MAA | Xenogeneic DNA vaccine, HMW-MAA fragment | Murine melanoma, renal carcinoma, Her-2 transgenic mice | Ab, CTL | HMW-MAA (2160-2225 aa) fragment fused to LLO | Maciag PC et al., 2008 [ | |
PDGFRβ | Autologous DNA vaccine, full-length | Murine colon, breast, lung carcinoma | CTL | Also, targets activated fibroblasts | Kaplan CD et al., 2006 [ | |
SURVIVIN | Xenogeneic DNA vaccine | Murine melanoma | Electroporation, ID | CTL | Human survivin vaccine | Lladser A et al., 2010 [ |
Survivin/CCL21 DNA vaccine | Murine lung carcinoma | CTL | Mouse survivin; no effect on wound closure or fertility | Xiang R et al., 2005 [ | ||
GRP | Recombinant chimeric HSP-65 -GRP6 fusion protein | Murine breast carcinomas | SC | Ab, CTL | 6 tandem repeats of GRP(18-27 aa) fused to HSP-65 | Guojun W et al., 2008 [ |
Chimeric-HSP65-GRP6 DNA Vaccine | Murine melanoma | "Naked" DNA, IM | Ab | chimera also includes tetanus toxoid and HSP70 fragments; | Fang J et al., 2009 [ | |
LEGUMAIN | Allogeneic DNA vaccine | Murine non-small lung, colon and breast cancers | CTL | Mutant polyubiquitin incorporated | Luo Y et al., 2006 | |
Autologous DNA minigene | Murine breast carcinoma | CTL | Angiogenesis inhibited more 90%; H-2K vaccine more potent than H-2D | Lewen S et al., 2008 [ | ||
MMP-2 | Xenogeneic full-length MMP-2 DNA vaccine | Murine fibrosarcoma, hepatoma, lung carcinoma | "Naked" DNA, IM | Ab | Chicken MMP-2 | Su JM et al., 2003 [ |
β3 Integrin | Xenogeneic β3 DNA vaccine | Murine fibrosarcoma, mammary carcinoma | "Naked" DNA, IM | Ab | Chicken β3 ligand binding domain | Lou YY et al., 2002 [ |
Besides tumor cells [41], dendritic cells (DC) pulsed with peptide/protein epitopes (or DNA encoding these epitopes) have also been employed successfully to vaccinate animals against tumor endothelial antigens [42, 43]. DC process and present antigens to T and B cells and produce cytokines and chemokines which in turn activate NK cells [44]. DC-based therapies involve modification with pulsed (loaded) defined peptides, whole protein lysate, and/or transfected DNA or RNA [42, 43]. An interesting anti-cancer and anti-angiogenic approach was the use of a VEGFR2-loaded DCs that led to greater than 80% reduction in lung metastases of two different tumor models [45]. Different forms of nucleic acids have also been used for angiogenic peptides and proteins. In addition to peptides, proteins, and recombinant DNA, mRNA is another promising strategy to enhance cellular immunity [46]. For example, antitumor synergy was observed when dendritic cells were transfected with mRNA from two receptors (VEGFR-2 and Tie2) that are highly expressed on tumor endothelial cells [47]. Varying the routes of pulsed DC administration may also affect the efficacy of tumor vaccines. Pellegatta and colleagues determined that glioblastomas regressed significantly more when mice received both intratumoral and subcutaneous pulsed DC injections compared to those which received subcutaneous injections [48].
Although most authors have not compared different carrier systems with one another, it is evident that the carrier system and route of administration are critical for the success of the vaccine in animal models and in human clinical trials [49]. We have already discussed differences in the immune response to survivin based on whether electroporation or direct injection of DNA was used. In addition, when Lai et al. compared three different delivery approaches (gene gun with non-coated particles, gene gun with coated gold particles, and intramuscular injection) for the EGFR plasmid vaccine, the gene-gun with non-coated particle vaccine had the greatest cytotoxic T-lymphocyte (CTL) response and anti-tumor response [49]. Interestingly, the CD4+ response and the levels of EGFR-specific antibodies were much greater with the coated gold particle method. The required robust immune response to overcome self-tolerance will no doubt eliminate several carriers, and perhaps autoimmunity will eliminate other carriers. Which of the carriers can be translated successfully from the animal models to humans remains to be determined. The delivery vehicle and the immune-adjuvant will likely be as important as the selected angiogenic antigen to obtain a successful tumor response in humans.
Approaches for Anti-Angiogenic Vaccines
A goal of vaccination in anti-angiogenic therapies targeting tumors is to break immune tolerance to self-antigens and induce specific, strong, and persisting immune response leading to eradication of cancer. Complex networks created by several immune-competent cells such as dendritic cells, B cells, cytotoxic CD8+ T, CD4+ T-helper, and NK cells in combination with cytokines, chemokines and other immune mediators are required for effective vaccines and immune reactions against cancer (Figure 1). Two anti-angiogenic vaccine approaches have shown promising results in reducing tumor growth and/or metastases: endothelial cell vaccines that demonstrate antitumor activity and vaccines targeting specific angiogenic targets.
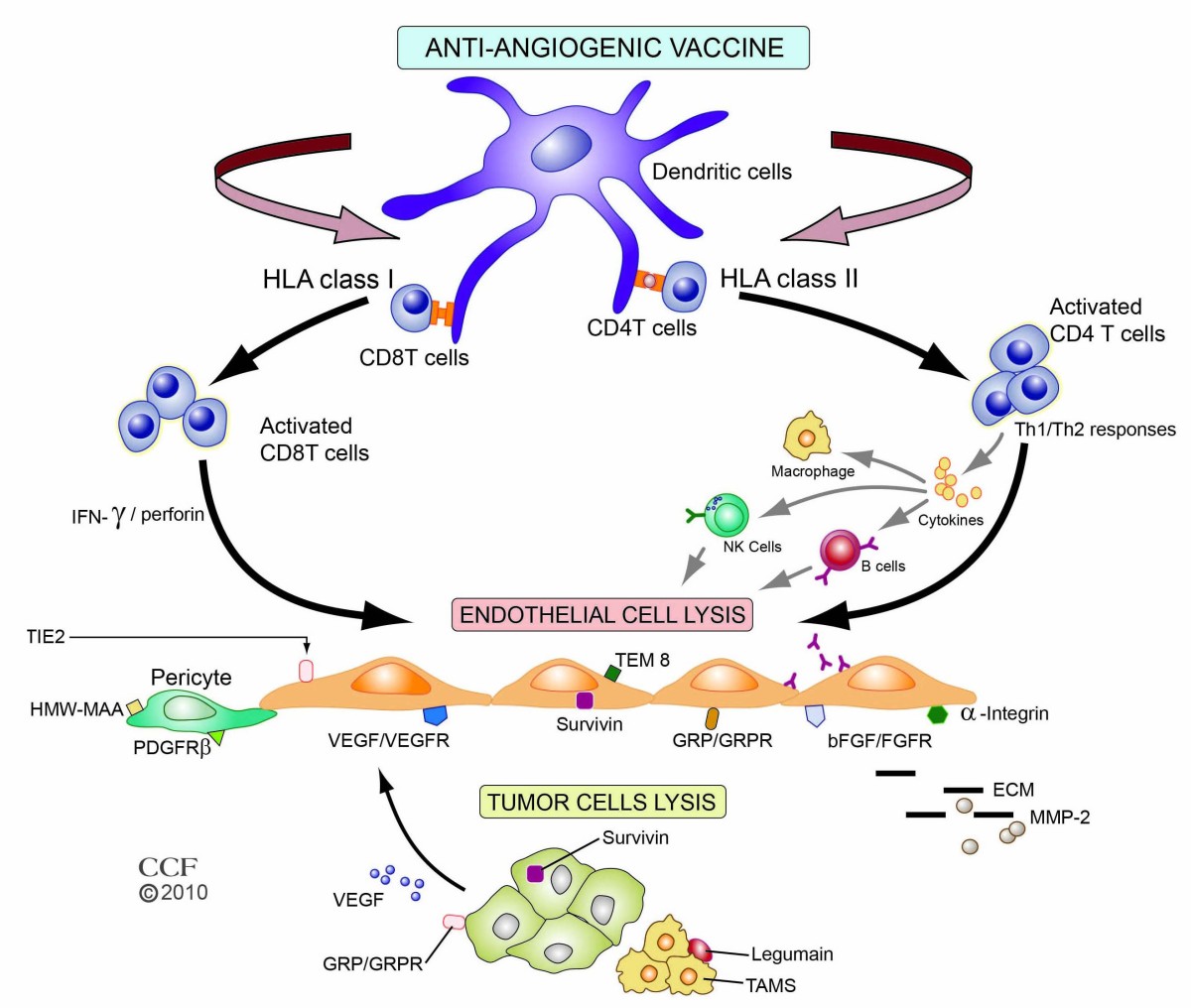
Figure 1
Figure 1 caption
Major mechanistic immune pathways of anti-angiogenic vaccines and their targets. Vaccine antigens are processed by antigen processing cells such as dendritic cells and presented to T cells. Depending on the antigen, the route of administration, and the vector, peptide presentation to either major histocompatibility complex (MHC) class I or II occurs, with subsequent interaction with T-cell receptors on CD4+ or CD8+ cells. Cytotoxic CD8+ T cells recognize and lyse tumor endothelial cells directly by perforin-mediated and Fas-mediated cytotoxic mechanisms. CD4+ T-helper cells, through release of different cytokines, can induce Th1 or Th2 responses that stimulate B-cells to produce antibodies and/or activate NK cells and macrophages to inhibit tumor endothelium. Representative targets related to endothelial and cancer cells and their environment for anti-angiogenic vaccines are depicted. Reprinted with permission, Cleveland Clinic Center for Medical Art & Photography © 2010. All Rights Reserved.
I. Endothelial Cell Vaccines
II. Vaccines Expressing Defined Targets
Defined endothelial vaccines are based on specific targets and include peptides and nucleic acids (DNA or RNA) that encode these peptides (see Table 1, Figure 2). Suitable angiogenic targets in tumors may be receptors/markers on endothelial cells or alternatively, may be growth factors secreted by cells other than endothelial cells. To date, there has been no target or epitope that is completely specific for tumor endothelial cells. For example, TEM8, one of the more specific tumor endothelial cell markers identified thus far, was originally found in the tumor vasculature and the developing embryo, but it has since been found on cell surfaces of melanomas, breast cancers, and dendritic cells. Despite the overlap in this system, we think that classification of angiogenic vaccines based on preponderance of their targets within most tumors may be useful. As a result, we have divided tumor endothelial vaccines with defined targets into three classes: 1) growth factors/receptors or epitopes that are primarily associated with growth of tumor endothelial cells; 2) growth factors/receptors or epitopes that promote growth of pericytes; and 3) proteins/growth factors/receptors that enhance both tumor and endothelial cell growth or survival. The growth factors were classified, not on their cells of origin, but on the location of their receptors.
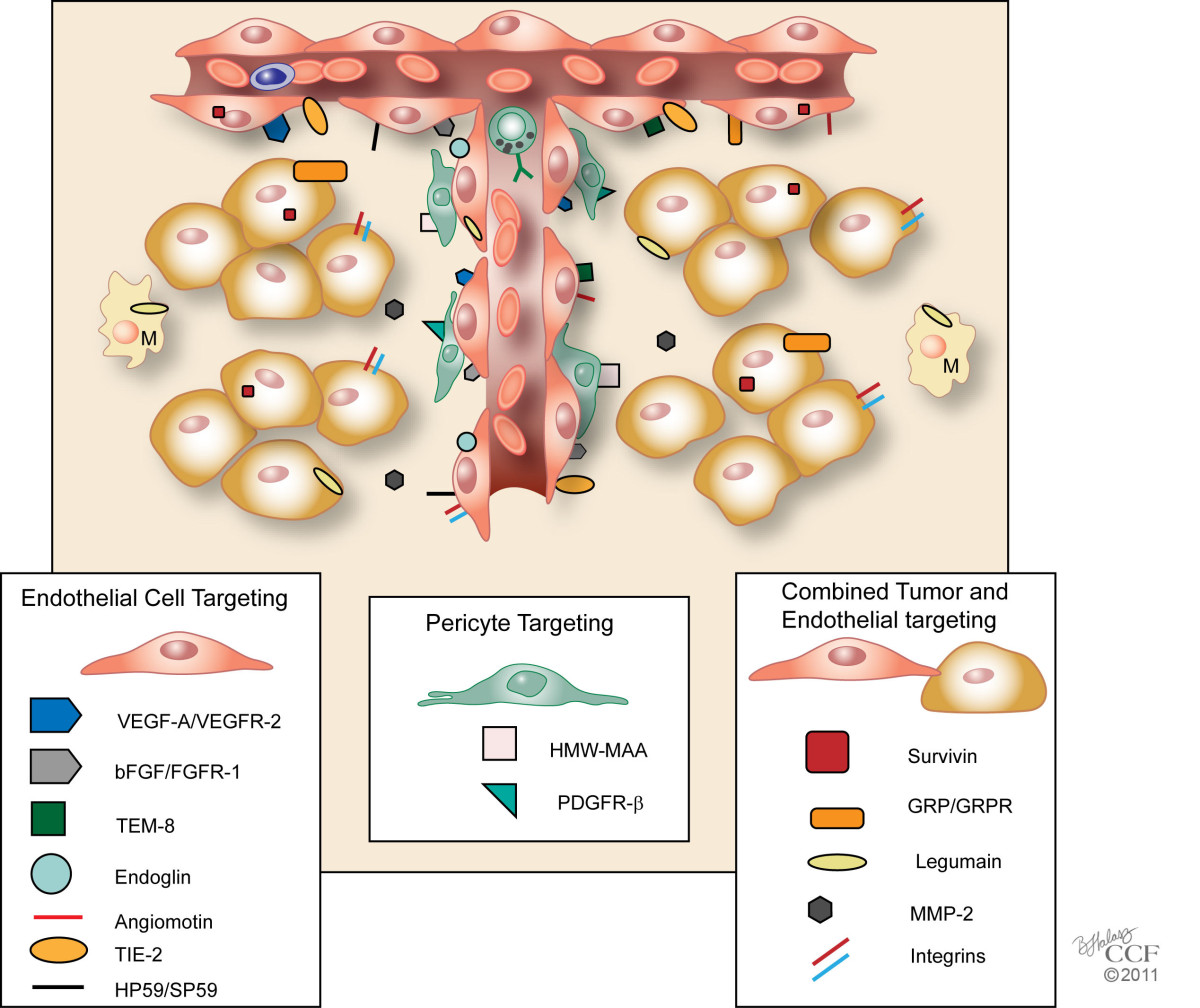
Figure 2
Figure 2 caption
Tumor Endothelial Vaccines with Defined Targets. Schematic model of a tumor and its angiogenic vessels are shown with targets of tumor endothelial vaccines. These vaccines may be classified on their specific targets 1) that are primarily associated with tumor endothelial cells, 2) that promote growth of pericytes, or 3) that enhance both tumor and endothelial cell growth or survival. Growth factors were classified based on the location of their receptors. M, Macrophage. Reprinted with permission, Cleveland Clinic Center for Medical Art & Photography © 2011. All Rights Reserved.
Conclusion
Significant resources including numerous pre-clinical and clinical studies have been devoted to the development of tumor vaccines. Thus far, these results have progressed and culminated in the approval a vaccine targeting advanced prostate cancer (Provenge). Although the benefits with this FDA-approved vaccine are modest, further improved vaccine versions are no doubt on the horizon and will aid with other vaccines approaches, including those against the tumor endothelium. Compared to tumor vaccines, the number of varied approaches for tumor endothelial vaccines is relatively limited and is currently restricted to pre-clinical experiments and primarily phase I trials.
As discussed in this review, several studies with tumor endothelial vaccines show anti-tumor efficacy in both transplantable and transgenic tumor-bearing animal models. Of particular note were the bacteria-based vaccines that showed marked anti-tumor response with varied anti-angiogenic genes and few if any side effects. Nevertheless, major obstacles still remain, including identification and validation of specific targets on the tumor endothelium, inhibition of local suppression mechanisms, and boosting anti-tumor immunity through NK cells [132]. Thus far, there have been few data from the endothelial vaccine studies on the various T-cell subtypes, including regulatory T-cells or myeloid derived suppressor cells [25, 133, 134], and comparison of T-cell subtypes in the tumor and peripheral tissues may be useful in development of more effective vaccines. Other immune cells such as NK cells have not yet shown a direct role in augmenting the efficacy of tumor endothelial vaccines [64, 87, 94, 130], but more research examining interactions among NK cells, dendritic cells, and immunomodulatory agents is needed [132, 135, 136].
Since tumor angiogenesis is a complex process, targeting a single epitope is unlikely to be successful. In many cases, the treated tumor adapts and finds alternative mechanisms of tumorigenesis eventually leading to resistance to therapy. Thus, combinations of anti-angiogenic vaccines with existing chemotherapy or immunomodulatory therapies offer interesting and exciting possibilities. For example, as discussed previously in this review, combinatory treatments between vaccines and IL-12, GM-CSF, CCL21, or β-defensins markedly increased the immune response toward tumor endothelial cells [39, 41, 62, 64]. Nevertheless, these co-stimulatory therapies have been used sparingly and other commonly used cytokines such as IL-2, IFN α or β [137–139] have not been co-administered or transfected into immune and/or endothelial cells to augment vaccine efficacy. Moreover, considerable more research is needed to determine the optimal co-stimulatory therapy to be administered with the vaccine for the different delivery methods.
Another consideration in developing anti-angiogenic vaccines is their potential for causing complications. Cross-reactivity between tumor and non-tumor disease tissues due to tumor endothelial vaccine may result in reduced compensatory biological processes. The classic tumor endothelial target is VEGFR2 up-regulated not only in the endothelial vessels of tumors but also in healing wounds and hypoxic cardiac tissues. Interestingly, at least with 4 vaccine studies (VEGFR2, VEGF-A, bFGF, survivin/CCL21) tumor angiogenesis was markedly inhibited, but these vaccines did not interfere with normal physiologic processes in several studies [32, 35, 47, 76, 77]. The mechanism whereby tolerance to self-angiogenic antigens in tumors but not in normal angiogenic processes is broken remains unknown. It has been suggested that differences in breaking self-angiogenic antigen tolerance between tumors and normal physiological processes may be based on the difference of their vascular organization [32, 140]; determining whether or not this is the mechanism for this difference will require further study.
To ensure effective tumor eradication and reduce autoimmune side effects, intensive efforts are still needed to identify additional targets specific to the tumor endothelium. One such study recently found highly specific and expressed markers of the tumor endothelium that were not expressed in quiescent blood vessels or physiologic angiogenesis [13]. Nevertheless, the efficacy of vaccines against these new markers has not yet been determined. Alternatively, finding tissue-specific vascular targets (e.g., prostate) may enable development of tumor endothelial vaccines with acceptable side effects [141, 142]. There is also the possibility that tailored endothelial vaccines may be developed based on specific endothelial epitopes associated with certain tumors [143]. As new anti-angiogenic targets are discovered, we anticipate that promising new therapeutic approaches are on the horizon.
Acknowledgements
The authors thank Drs. Pamela Talalay and Paul Anthony for their helpful suggestions and careful reading of this manuscript. This work was supported by the National Institutes of Health (R01-CA136938).
Authors’ original submitted files for images
Below are the links to the authors’ original submitted files for images.
Authors’ original file for figure 1
Authors’ original file for figure 2
References
- Clinical experience with angiogenesis signaling inhibitors: focus on vascular endothelial growth factor (VEGF) blockers. Cancer Control. 2002;9:36-44.
- Hypoxia-inducible factors: central regulators of the tumor phenotype. Curr Opin Genet Dev. 2007;17:71-77.
- Hypoxia-inducible factors, hypoxia, and tumor angiogenesis. Curr Opin Hematol. 2006;13:169-174.
- Search for Clinical Trials-National Cancer Institute. [http://www.cancer.gov/clinicaltrials/search]
- Tumor vasculature as target for therapeutic intervention. Expert Opin Investig Drugs. 2010;19:1321-1338.
- Blocking VEGFR-3 suppresses angiogenic sprouting and vascular network formation. Nature. 2008;454:656-660.
- Angiopoietin-2: development of inhibitors for cancer therapy. Curr Oncol Rep. 2009;11:111-116.
- Targeting tumor angiogenesis with gene therapy. Mol Genet Metab. 2001;74:120-127.
- Off-tumor target--beneficial site for antiangiogenic cancer therapy?. Nat Rev Clin Oncol. 2010;7:604-608.
- Angiogenesis: What can it offer for future medicine?. Exp Cell Res. 2010;316:1304-1308.
- Optimizing the delivery of cancer drugs that block angiogenesis. Sci Transl Med. 2010;2:15ps13-.
- Genes expressed in human tumor endothelium. Science. 2000;289:1197-1202.
- Genes that distinguish physiological and pathological angiogenesis. Cancer Cell. 2007;11:539-554.
- Antiangiogenic therapy of experimental cancer does not induce acquired drug resistance. Nature. 1997;390:404-407.
- Vascular remodeling marks tumors that recur during chronic suppression of angiogenesis. Mol Cancer Res. 2004;2:36-42.
- Tumor-associated endothelial cells with cytogenetic abnormalities. Cancer Res. 2004;64:8249-8255.
- A new perspective on tumor endothelial cells: unexpected chromosome and centrosome abnormalities. Cancer Res. 2005;65:2507-2510.
- Tumour vascularization via endothelial differentiation of glioblastoma stem-like cells. Nature. 2010;468:824-828.
- Glioblastoma stem-like cells give rise to tumour endothelium. Nature. 2010;468:829-833.
- Fighting cancer by attacking its blood supply. Sci Am. 1996;275:150-154.
- Antiangiogenic Therapy. Principles and Practice of Oncology. 1997.:3075-3085.
- Integrating the anti-VEGF-A humanized monoclonal antibody bevacizumab with chemotherapy in advanced colorectal cancer. Clin Colorectal Cancer. 2004;4(Suppl 2):S62-68.
- The use of bevacizumab in colorectal, lung, breast, renal and ovarian cancer: where does it fit?. Eur J Cancer. 2008;44:2350-2356.
- Targeting angiogenesis driven by vascular endothelial growth factors using antibody-based therapies. Cancer J. 2008;14:170-177.
- Targeting the immune system in cancer. Curr Pharm Biotechnol. 2009;10:166-184.
- Vaccines against human carcinomas: strategies to improve antitumor immune responses. J Biomed Biotechnol. 2010;2010:380697-.
- DNA vaccines: developing new strategies against cancer. J Biomed Biotechnol. 2010;2010:174378-.
- Whole tumor antigen vaccines. Semin Immunol. 2010;22:132-143.
- DNA vaccination strategies for anti-tumour effective gene therapy protocols. Cancer Immunol Immunother. 2010;59:1583-1591.
- DNA vaccines designed to inhibit tumor growth by suppression of angiogenesis. Int Arch Allergy Immunol. 2004;133:295-304.
- Harnessing Listeria monocytogenes to target tumors. Cancer Biol Ther. 2010;9:257-265.
- An anti-vascular endothelial growth factor receptor 2/fetal liver kinase-1 Listeria monocytogenes anti-angiogenesis cancer vaccine for the treatment of primary and metastatic Her-2/neu+ breast tumors in a mouse model. J Immunol. 2009;182:5537-5546.
- Cancer immunotherapy targeting the high molecular weight melanoma-associated antigen protein results in a broad antitumor response and reduction of pericytes in the tumor vasculature. Cancer Res. 2008;68:8066-8075.
- A novel DNA vaccine encoding PDGFRbeta suppresses growth and dissemination of murine colon, lung and breast carcinoma. Vaccine. 2006;24:6994-7002.
- A DNA vaccine targeting survivin combines apoptosis with suppression of angiogenesis in lung tumor eradication. Cancer Res. 2005;65:553-561.
- Use of attenuated bacteria as delivery vectors for DNA vaccines. Expert Rev Vaccines. 2007;6:97-110.
- Intradermal DNA electroporation induces survivin-specific CTLs, suppresses angiogenesis and confers protection against mouse melanoma. Cancer Immunol Immunother. 2010;59:81-92.
- A DNA vaccine targeting angiomotin inhibits angiogenesis and suppresses tumor growth. Proc Natl Acad Sci USA. 2006;103:9208-9213.
- Naked DNA immunization as an approach to target the generic tumor antigen survivin induces humoral and cellular immune responses in mice. Immunobiology. 2006;211:11-27.
- Survivin DNA vaccine generated specific antitumor effects in pancreatic carcinoma and lymphoma mouse models. Vaccine. 2007;25:7955-7961.
- Combined allogeneic tumor cell vaccination and systemic interleukin 12 prevents mammary carcinogenesis in HER-2/neu transgenic mice. J Exp Med. 2001;194:1195-1205.
- Active antitumor immunity elicited by vaccine based on recombinant form of epidermal growth factor receptor. J Immunother. 2005;28:236-244.
- Rationale for antiangiogenic cancer therapy with vaccination using epitope peptides derived from human vascular endothelial growth factor receptor 2. Cancer Res. 2005;65:4939-4946.
- Dendritic-cell immunotherapy: from ex vivo loading to in vivo targeting. Nature Reviews Immunology. 2007;7:790-802.
- Active immunization against the vascular endothelial growth factor receptor flk1 inhibits tumor angiogenesis and metastasis. J Exp Med. 2002;195:1575-1584.
- Induction of tumor-specific cytotoxic T lymphocytes in cancer patients by autologous tumor RNA-Transfected dendritic cells. Annals of Surgery. 2002;235:540-549.
- Synergy between tumor immunotherapy and antiangiogenic therapy. Blood. 2003;102:964-971.
- Intra-tumoral dendritic cells increase efficacy of peripheral vaccination by modulation of glioma microenvironment. Neuro Oncol. 2010;12:377-388.
- The effects of DNA formulation and administration route on cancer therapeutic efficacy with xenogenic EGFR DNA vaccine in a lung cancer animal model. Genet Vaccines Ther. 2009;7:2-.
- Immunotherapy of tumors with xenogeneic endothelial cells as a vaccine. Nat Med. 2000;6:1160-1166.
- Vaccination with autologous endothelium inhibits angiogenesis and metastasis of colon cancer through autoimmunity. Cancer Science. 2004;95:85-90.
- Induction of anti-tumor immunity in mice using a syngeneic endothelial cell vaccine. Anticancer Res. 2003;23:1165-1172.
- Inhibition of B16 melanoma growth and metastasis in C57BL mice by vaccination with a syngeneic endothelial cell line. Journal of Experimental & Clinical Cancer Research. 2009;28:13-.
- Pilot study of anti-angiogenic vaccine using fixed whole endothelium in patients with progressive malignancy after failure of conventional therapy. European Journal of Cancer. 2008;44:383-390.
- Vaccination with xenogeneic tumor endothelial proteins isolated in situ inhibits tumor angiogenesis and spontaneous metastasis. Int J Cancer. 2009;125:124-132.
- Failure of blood-island formation and vasculogenesis in Flk-1-deficient mice. Nature. 1995;376:62-66.
- Antiangiogenic and antivascular therapy for cancer. Curr Opin Pharmacol. 2001;1:378-384.
- Immunogene therapy of tumors with vaccine based on Xenopus homologous vascular endothelial growth factor as a model antigen. Proc Natl Acad Sci USA. 2001;98:11545-11550.
- A DNA vaccine against VEGF receptor 2 prevents effective angiogenesis and inhibits tumor growth. Nat Med. 2002;8:1369-1375.
- Combination of low-dose gemcitabine and recombinant quail vascular endothelial growth factor receptor-2 as a vaccine induces synergistic antitumor activities. Oncology. 2005;69:81-87.
- T cell-mediated suppression of angiogenesis results in tumor protective immunity. Blood. 2005;106:2026-2032.
- Identification of H-2Db-specific CD8+ T-cell epitopes from mouse VEGFR2 that can inhibit angiogenesis and tumor growth. J Immunother. 2006;29:32-40.
- FLK-1-based minigene vaccines induce T cell-mediated suppression of angiogenesis and tumor protective immunity in syngeneic BALB/c mice. Vaccine. 2007;25:1409-1415.
- Immunity against tumor angiogenesis induced by a fusion vaccine with murine beta-defensin 2 and mFlk-1. Clin Cancer Res. 2007;13:6779-6787.
- Anti-tumor effects of a human VEGFR-2-based DNA vaccine in mouse models. Genet Vaccines Ther. 2009;7:10-.
- Phase I clinical trial using peptide vaccine for human vascular endothelial growth factor receptor 2 in combination with gemcitabine for patients with advanced pancreatic cancer. Cancer Sci. 2010;101:433-439.
- Orally administered DNA vaccine delivery by attenuated Salmonella typhimurium targeting fetal liver kinase 1 inhibits murine Lewis lung carcinoma growth and metastasis. Biol Pharm Bull. 2010;33:174-182.
- Beta-defensins: linking innate and adaptive immunity through dendritic and T cell CCR6. Science. 1999;286:525-528.
- Toll-like receptor 4-dependent activation of dendritic cells by beta-defensin 2. Science. 2002;298:1025-1029.
- Cell-retained isoforms of vascular endothelial growth factor (VEGF) are correlated with poor prognosis in osteosarcoma. Eur J Cancer. 1999;35:1089-1093.
- Expression of cell-associated isoform of vascular endothelial growth factor 189 and its prognostic relevance in non-small cell lung cancer. Int J Oncol. 1998;12:541-544.
- [Antitumor effect of recombinant T7 phage vaccine expressing xenogenic vascular endothelial growth factor on Lewis lung cancer in mice]. Ai Zheng. 2006;25:1221-1226.
- VEGF kinoid vaccine, a therapeutic approach against tumor angiogenesis and metastases. Proc Natl Acad Sci USA. 2007;104:2837-2842.
- VEGF-A splicing: the key to anti-angiogenic therapeutics?. Nat Rev Cancer. 2008;8:880-887.
- VEGF 165 b, an antiangiogenic VEGF-A isoform, binds and inhibits bevacizumab treatment in experimental colorectal carcinoma: balance of pro- and antiangiogenic VEGF-A isoforms has implications for therapy. Br J Cancer. 2008;98:1366-1379.
- Administration of a liposomal FGF-2 peptide vaccine leads to abrogation of FGF-2-mediated angiogenesis and tumor development. Vaccine. 2000;19:1294-1303.
- Generation of a specific immunological response to FGF-2 does not affect wound healing or reproduction. Immunopharmacol Immunotoxicol. 2004;26:29-41.
- Inhibition of tumor growth with a vaccine based on xenogeneic homologous fibroblast growth factor receptor-1 in mice. J Biol Chem. 2003;278:21831-21836.
- TEM8 interacts with the cleaved C5 domain of collagen alpha 3(VI). Cancer Res. 2004;64:817-820.
- Cell surface tumor endothelial markers are conserved in mice and humans. Cancer Res. 2001;61:6649-6655.
- Tumor endothelial marker 8 expression levels in dendritic cell-based cancer vaccines are related to clinical outcome. Cancer Immunol Immunother. 2010;59:27-34.
- Tumor endothelial marker 8 enhances tumor immunity in conjunction with immunization against differentiation Ag. Cytotherapy. 2007;9:23-34.
- DNA vaccine against tumor endothelial marker 8 inhibits tumor angiogenesis and growth. J Immunother. 2009;32:486-491.
- Prognostic significance of angiogenesis evaluated by CD105 expression compared to CD31 in 905 breast carcinomas: correlation with long-term patient outcome. Int J Oncol. 2004;24:1197-1204.
- Association of serum endoglin with metastasis in patients with colorectal, breast, and other solid tumors, and suppressive effect of chemotherapy on the serum endoglin. Clin Cancer Res. 2001;7:524-532.
- Anti-cancer therapies targeting the tumor stroma. Cancer Immunol Immunother. 2008;57:1-17.
- Active immunotherapy of tumors with a recombinant xenogeneic endoglin as a model antigen. Eur J Immunol. 2004;34:2012-2021.
- Combination of low-dose cisplatin and recombinant xenogeneic endoglin as a vaccine induces synergistic antitumor activities. Int J Cancer. 2004;112:701-706.
- Angiomotin: an angiostatin binding protein that regulates endothelial cell migration and tube formation. J Cell Biol. 2001;152:1247-1254.
- Angiomotin regulates endothelial cell-cell junctions and cell motility. J Biol Chem. 2005;280:34859-34869.
- Angiomotin and angiomotin like proteins, their expression and correlation with angiogenesis and clinical outcome in human breast cancer. BMC Cancer. 2006;6:16-.
- Expression of Tie2/Tek in breast tumour vasculature provides a new marker for evaluation of tumour angiogenesis. Br J Cancer. 1998;77:51-56.
- Antiangiogenic gene therapy targeting the endothelium-specific receptor tyrosine kinase Tie2. Proc Natl Acad Sci USA. 1998;95:8829-8834.
- Immunotherapy of tumors with protein vaccine based on chicken homologous Tie-2. Clin Cancer Res. 2006;12:1813-1819.
- Identification of an HLA-A*0201 cytotoxic T lymphocyte epitope specific to the endothelial antigen Tie-2. Int J Cancer. 2004;110:245-250.
- Identification of a novel membrane protein, HP59, with therapeutic potential as a target of tumor angiogenesis. Clin Cancer Res. 2001;7:4182-4194.
- Studies on group B beta-hemolytic Streptococcus. II. Effects on pulmonary hemodynamics and vascular permeability in unanesthetized sheep. Pediatr Res. 1981;15:899-904.
- Isolation and identification of the group B streptococcal toxin CM101 from infants with sepsis. J Pediatr. 2000;137:338-344.
- Regulatory mechanisms for the expression and activity of platelet-derived growth factor receptor. Acta Biochim Pol. 2003;50:647-658.
- PDGF receptors-mediators of autocrine tumor growth and regulators of tumor vasculature and stroma. Cytokine Growth Factor Rev. 2004;15:275-286.
- PDGFRbeta+ perivascular progenitor cells in tumours regulate pericyte differentiation and vascular survival. Nat Cell Biol. 2005;7:870-879.
- Survivin minigene DNA vaccination is effective against neuroblastoma. Int J Cancer. 2009;125:104-114.
- Gastrin-releasing peptide: in vivo and in vitro growth effects on an acinar pancreatic carcinoma. Cancer Res. 1992;52:3726-3732.
- Gastrin-releasing peptide receptor-mediated autocrine growth in squamous cell carcinoma of the head and neck. J Natl Cancer Inst. 2002;94:375-383.
- Gastrin-releasing peptide is a growth factor for human neuroblastomas. Ann Surg. 2002;235:621-629.
- Bombesin-like peptides can function as autocrine growth factors in human small-cell lung cancer. Nature. 1985;316:823-826.
- Selective stimulation of small cell lung cancer clonal growth by bombesin and gastrin-releasing peptide. Cancer Res. 1987;47:821-825.
- Stimulation by bombesin and inhibition by bombesin/gastrin-releasing peptide antagonist RC-3095 of growth of human breast cancer cell lines. Cancer Res. 1992;52:4545-4547.
- Gastrin releasing peptide GRP(14-27) in human breast cancer cells and in small cell lung cancer. Breast Cancer Res Treat. 1991;19:119-128.
- Gastrin releasing peptide and gastrin releasing peptide receptor expression in gastrointestinal carcinoid tumours. J Clin Pathol. 2004;57:189-192.
- Gastrin-releasing peptide (GRP) induces angiogenesis and the specific GRP blocker 77427 inhibits tumor growth in vitro and in vivo. Oncogene. 2005;24:4106-4113.
- Bombesin stimulates nuclear factor kappa B activation and expression of proangiogenic factors in prostate cancer cells. Cancer Res. 2003;63:3495-3502.
- Bombesin induces angiogenesis and neuroblastoma growth. Cancer Lett. 2007;253:273-281.
- Gastrin-releasing peptide receptor as a molecular target in experimental anticancer therapy. Ann Oncol. 2007;18:1457-1466.
- A novel vaccine targeting gastrin-releasing peptide: efficient inhibition of breast cancer growth in vivo. Endocr Relat Cancer. 2008;15:149-159.
- Specific antibodies elicited by a novel DNA vaccine targeting gastrin-releasing peptide inhibit murine melanoma growth in vivo. Clin Vaccine Immunol. 2009;16:1033-1039.
- Overexpression of legumain in tumors is significant for invasion/metastasis and a candidate enzymatic target for prodrug therapy. Cancer Res. 2003;63:2957-2964.
- Legumain expression in relation to clinicopathologic and biological variables in colorectal cancer. Clin Cancer Res. 2005;11:2293-2299.
- Targeting tumor-associated macrophages as a novel strategy against breast cancer. J Clin Invest. 2006;116:2132-2141.
- A Legumain-based minigene vaccine targets the tumor stroma and suppresses breast cancer growth and angiogenesis. Cancer Immunol Immunother. 2008;57:507-515.
- Matrix metalloproteinase inhibition as a novel anticancer strategy: a review with special focus on batimastat and marimastat. Pharmacol Ther. 1997;75:69-75.
- Combination therapy including a gelatinase inhibitor and cytoxic agent reduces local invasion and metastasis of murine Lewis lung carcinoma. Cancer Research. 1996;56:715-718.
- Active immunogene therapy of cancer with vaccine on the basis of chicken homologous matrix metalloproteinase-2. Cancer Res. 2003;63:600-607.
- The Trouble with Inhibitors. Signalling scissors: new perspectives on proteases; Brescia, Italy. 2003.
- MMP inhibitors: glimmers of hope amidst clinical failures. Nat Med. 2002;8:95-.
- Towards third generation matrix metalloproteinase inhibitors for cancer therapy. Br J Cancer. 2006;94:941-946.
- Tumour microenvironment - opinion: validating matrix metalloproteinases as drug targets and anti-targets for cancer therapy. Nat Rev Cancer. 2006;6:227-239.
- Phase I and pharmacokinetic study of continuous twice weekly intravenous administration of Cilengitide (EMD 121974), a novel inhibitor of the integrins alphavbeta3 and alphavbeta5 in patients with advanced solid tumours. Eur J Cancer. 2003;39:917-926.
- Immunogene therapy of tumors with a vaccine based on the ligand-binding domain of chicken homologous integrin beta3. Immunol Invest. 2002;31:51-69.
- Immunotherapy of tumors with vaccine based on quail homologous vascular endothelial growth factor receptor-2. Blood. 2003;102:1815-1823.
- Bevacizumab in the Treatment of Patients with Advanced Breast Cancer: Where have We Landed?. Ther Adv Med Oncol. 2010;2:331-342.
- Human NK cells lyse organ-specific endothelial cells: analysis of adhesion and cytotoxic mechanisms. J Immunol. 2005;174:5573-5582.
- Induction of immune response against NY-ESO-1 by CHP-NY-ESO-1 vaccination and immune regulation in a melanoma patient. Cancer Immunol Immunother. 2008;57:1429-1437.
- Myeloid-derived suppressor cells: more mechanisms for inhibiting antitumor immunity. Cancer Immunol Immunother. 2010;59:1593-1600.
- The anti-angiogenic activity of IL-12 is increased in iNOS-/- mice and involves NK cells. J Mol Med. 2010;88:775-784.
- NK cell activation by dendritic cell vaccine: a mechanism of action for clinical activity. Cancer Immunol Immunother. 2006;55:1122-1131.
- Inhibition of angiogenesis by interferons: effects on tumor- and lymphocyte-induced vascular responses. Cancer Res. 1987;47:5155-5161.
- Synergistic effect of retinoids and interferon alpha on tumor-induced angiogenesis: anti-angiogenic effect on HPV-harboring tumor-cell lines. Int J Cancer. 1994;57:81-85.
- Interferon-alpha-mediated down-regulation of angiogenesis-related genes and therapy of bladder cancer are dependent on optimization of biological dose and schedule. Clin Cancer Res. 1999;5:2726-2734.
- Gap junction communication between autologous endothelial and tumor cells induce cross-recognition and elimination by specific CTL. J Immunol. 2009;182:2654-2664.
- Targeting the prostate for destruction through a vascular address. Proc Natl Acad Sci USA. 2002;99:1527-1531.
- In vivo phage display and vascular heterogeneity: implications for targeted medicine. Curr Opin Chem Biol. 2002;6:399-404.
- Vascular homing peptides with cell-penetrating properties. Curr Pharm Des. 2005;11:3655-3660.