Deciphering the roles of macrophages in developmental and inflammation stimulated lymphangiogenesis
Vascular Cell. 2012;
Received: 3 July 2012 | Accepted: 13 July 2012 | Published: 3 September 2012
Vascular Cell ISSN: 2045-824X
Abstract
Lymphatic vessels share an intimate relationship with hematopoietic cells that commences during embryogenesis and continues throughout life. Lymphatic vessels provide a key conduit for immune cell trafficking during immune surveillance and immune responses and in turn, signals produced by immune lineage cells in settings of inflammation regulate lymphatic vessel growth and activity. In the majority of cases, the recruitment and activation of immune cells during inflammation promotes the growth and development of lymphatic vessels (lymphangiogenesis) and enhances lymph flow, effects that amplify cell trafficking to local lymph nodes and facilitate the mounting of effective immune responses. Macrophages comprise a major, heterogeneous lineage of immune cells that, in addition to key roles in innate and adaptive immunity, perform diverse tasks important for tissue development, homeostasis and repair. Here, we highlight the emerging roles of macrophages in lymphangiogenesis, both during development and in settings of pathology. While much attention has focused on the production of pro-lymphangiogenic stimuli including VEGF-C and VEGF-D by macrophages in models of inflammation including cancer, there is ample evidence to suggest that macrophages provide additional signals important for the regulation of lymphatic vascular growth, morphogenesis and function.
Keywords
Lymphangiogenesis Macrophages Monocytes Development Inflammation VEGF-C VEGF-DThe many faces of macrophages
Macrophages encompass a phenotypically heterogeneous population of cells that play a rapidly expanding catalogue of roles during development, homeostasis and disease [1–3]. While perhaps best recognised for the key roles they fulfil in innate and adaptive immunity, macrophages (literally “large eaters”, due to their phagocytic capabilities) also provide apoptosis inducing stimuli important for tissue remodeling and maturation [4–6], cues that instruct organ patterning and morphogenesis [7–12] and signals important for tissue regeneration and repair [13–15].
Macrophage diversity is obvious both in the embryo and the adult. In the embryo, macrophage subtypes can be distinguished on the basis of differential expression of markers including lymphatic vessel endothelial hyaluronan receptor (LYVE-1) and the angiopoietin receptor Tie2 [16, 17]. In the adult, distinct populations of circulating monocytes are categorised as “inflammatory” or “resident” monocytes based on the expression of markers including Gr1/Ly6C and the chemokine receptors CX3CR1 and CCR2 [18]. Tie2 has been reported to mark a distinct lineage of monocytes, termed Tie2-expressing monocytes (TEMs), that are recruited to the tumor microenvironment where they promote the growth of new blood vessels [19]. In response to stimuli encountered, monocytes may be induced to differentiate into macrophage subtypes including classically activated, “M1” inflammatory macrophages or alternatively activated, “M2” regulatory and wound healing macrophages. There is little doubt, however, that the M1 and M2 classification oversimplifies the extent of macrophage heterogeneity [20, 21]. Mature, specialist macrophages found in adult tissues include Kupffer cells of the liver that clear spent erythrocytes from the circulation, microglia in the central nervous system that regulate neural development and osteoclasts in the bone marrow important for bone remodeling [1, 3].
Recent work has revealed that macrophages play key roles during vascular development; these include directing regression of the hyaloid blood vascular plexus [4, 5, 22], facilitating the anastomosis of sprouting blood vessels [10] and patterning the retinal vasculature [23, 24]. Furthermore, macrophages have been shown to promote neo-vascularization in settings of inflammation and wound repair by producing pro-angiogenic growth factors, chemokines and proteases [19, 25–27]. In this review, we focus on the roles that macrophages play in lymphatic vascular growth and development (lymphangiogenesis), both during development and in disease.
Developmental origins of macrophages and lymphatic vessels
The developmental origins of macrophage subsets are being progressively unravelled, with genetic lineage tracing studies providing answers to longstanding questions regarding progenitor cell origin and the differentiation potential of various monocyte/macrophage populations. Current data suggest that maternally derived macrophages are the first to appear in the mouse embryo at approximately embryonic day (E) 7.5 [28]. Subsequently, macrophages originating in the yolk sac (E8) and from definitive hematopoietic progenitor cells arising in the embryonic aorta-gonad-mesonephros (AGM) (E10.0) and foetal liver develop (E10.5-onwards) [28]. After birth, the major site of hematopoiesis is the bone marrow. Recent evidence supports the concept that mature macrophages in the adult are derived from distinct progenitor pools; microglia in the brain appear to be descendents of primitive myeloid progenitors that arise prior to E8 [29], while circulating monocytes that give rise to the majority of tissue macrophages are derived from definitive hematopoietic stem cells.
Construction of the lymphatic vasculature is initiated once the major arteries (dorsal aortae) and veins (cardinal veins) have been established in the embryo, originating from the cardinal veins following the onset of expression of the Prox1 transcription factor in a polarised population of venous endothelial cells at ~ E9.5 [30]. Prox1-positive lymphatic endothelial progenitor cells exit the cardinal veins via sprouting and ballooning mechanisms to form lymph sacs and the superficial lymphatic vascular plexus [30, 31], a process that is dependent on vascular endothelial growth factor C (VEGF-C) [32]. The initiation of Prox1 expression in lymphatic endothelial progenitor cells in the cardinal veins signifies lymphatic endothelial cell fate commitment and is dependent on the activity of Sox18 and CoupTFII transcription factors [33, 34]. Once established, the lymphatic vasculature regulates tissue fluid homeostasis, immune cell trafficking and the absorption of dietary fats [35, 36].
Work in a variety of vertebrate models has suggested that mesodermal cells, including those of the macrophage lineage, might contribute to genesis of the lymphatic vasculature during development by comprising a pool of lymphatic endothelial progenitor cells [37–40]. These studies have suggested that mesenchymal “lymphangioblasts” expressing both LEC (Prox1/LYVE-1) and macrophage markers (LYVE-1, CD45, F4/80) integrate to growing lymphatic vessels in the developing embryo [38, 40]. In contrast, work from others has concluded that the vast majority of embryonic lymphatic endothelial cells are derived from the venous progenitor pool, with no evidence to support the concept of monocytes or macrophages giving rise to lymphatic endothelial cells [17, 41]. These discrepancies may potentially be a result of differences between the vertebrate models employed in these studies, or of the techniques utilized to assess macrophage incorporation into lymphatic vessels (marker expression versus lineage tracing). In fact, embryonic LYVE-1-positive macrophages have been found to share an intimate spatial association with embryonic lymphatic vessels (Figure 1) and in some cases appear incorporated into the wall of developing lymphatic vessels, but lineage tracing studies in the mouse embryo have not detected Prox1 expression in LYVE-1-positive, myeloid derived cells [17]. This data suggests that these cells retain a macrophage identity even when resident in lymphatic vessels. Gene expression profiling of embryonic dermal LYVE-1-positive macrophages revealed a close resemblance to TEMs [16, 17], a population of macrophages that not only play key roles in tumor stimulated angiogenesis, but are important for blood vascular development [10, 16, 19, 42]. These data suggest that LYVE-1-positive macrophages may be important for morphogenesis or remodelling of the lymphatic vasculature. Studies utilising real time imaging will be required to discern whether LYVE-1-positive macrophages assume localisation in the walls of lymphatic vessels in order to perform an immune surveillance role, whether they transit through the lymphatic endothelium while patrolling the embryo, or whether they fulfil roles important for lymphatic vascular development.
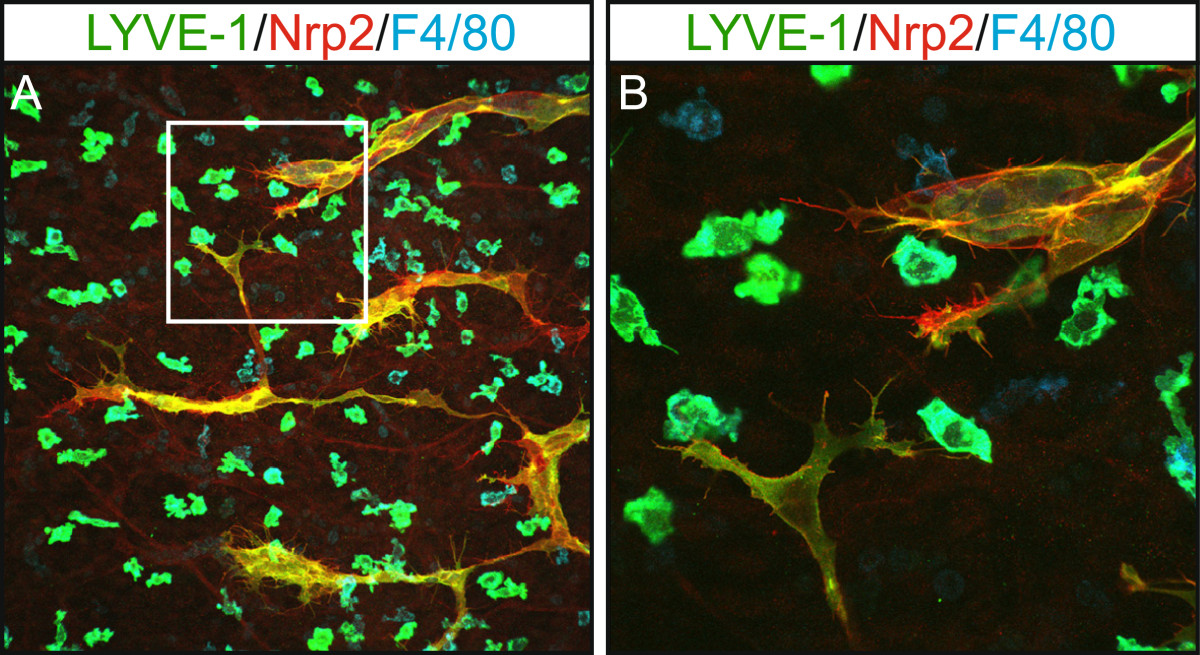
Figure 1
Figure 1 caption
LYVE-1 positive macrophages share an intimate association with growing lymphatic vessels in embryonic mouse skin. Whole mount immunostaining of E14.5 skin illustrating the close association of lymphatic vessel filopodia (Nrp2-positive, LYVE-1-positive) with dermal macrophages (F4/80-positive, LYVE-1-positive). Area boxed in panel A is shown at higher magnification in panel B.
Macrophages as a source of growth and patterning signals in developmental lymphangiogenesis
While it seems unlikely that monocytes/macrophages comprise a lymphatic endothelial progenitor cell pool during embryogenesis, recent work from a number of groups has identified an important role for macrophages in patterning the lymphatic vasculature. Akin to the role identified for macrophages in mediating the anastomosis of sprouting blood vessels in the embryonic brain [10] and postnatal retina [23], work from Kubota and colleagues suggested that LYVE-1-positive macrophages regulate density of the lymphatic vasculature in selected postnatal tissues [43]. Analysis of
Work from Bohmer and colleagues demonstrated that embryonic dermal macrophages expressing the tyrosine kinase Syk closely resemble TEMs and express high levels of pro-lymphangiogenic molecules including vascular endothelial growth factor C (VEGF-C), vascular endothelial growth factor D (VEGF-D), fibroblast growth factor 2 (FGF2), matrix metalloprotease 2 (MMP-2) and matrix metalloprotease 9 (MMP-9) [45]. In the absence of Syk, an increased number of these pro-lymphangiogenic monocytes/macrophages expressing elevated levels of growth factors and chemokines accumulated in skin and as a result,
Macrophages in inflammation and tumor-stimulated lymphangiogenesis
The roles played by macrophages in pathological lymphangiogenesis are rapidly gaining recognition. The induction of lymphangiogenesis during wound healing and in many pathological settings normally acts to resolve inflammation and edema; in models of infection and acute inflammation, increased lymphangiogenesis has been associated with elevated lymph flow, enhanced immune cell trafficking to draining lymph nodes and the resolution of tissue inflammation and edema [48–50]. While this is advantageous in the majority of settings, lymphangiogenesis induced following organ transplantation has been shown to aid alloimmunization and thereby promote the rejection of kidney, corneal and pancreatic islet transplants [51–53]. Understanding how lymphangiogenesis is regulated during inflammation thereby stands to advance the development of new therapeutics able to promote or ablate lymphangiogenesis dependent on the pathological setting.
Macrophages have been demonstrated to drive lymphangiogenesis in models of inflammation including bacterial infection [48, 49, 54], wound healing [55, 56], organ transplant [57, 58], rheumatoid arthritis [59], pancreatic islet inflammation/diabetes [60] and atopic dermatitis [61]. Macrophages also appear to be key players in salt-induced hypertension, where macrophage derived VEGF-C is important for inducing lymphangiogenesis as a buffering mechanism to deal with increased interstitial fluid accumulation [62]. At least two mechanisms by which cells of the monocyte/macrophage lineage promote neo-lymphangiogenesis have been proposed; trans-differentiation to lymphatic endothelial cells [55, 57, 63–65] and production of pro-lymphangiogenic stimuli including VEGF-C, VEGF-D and VEGF-A [48, 49, 54, 66–68] (Figure 2). The relative contribution that macrophages provide to inflammation-stimulated lymphangiogenesis has been illustrated in studies of macrophage deficient mice [43] and by the depletion of macrophages using clodronate liposomes [49, 67], c-fms inhibition [43] or VEGF-A inhibition [49, 67, 69]. VEGF-C and/or VEGF-D appear to be critical for the pro-lymphangiogenic activity of macrophages in the majority of models studied; blockade of VEGF-C and VEGF-D activity using soluble VEGFR-3 or VEGFR-3 neutralising antibodies has been demonstrated to inhibit macrophage driven lymphangiogenesis [48, 49, 52, 68]. Recent work has suggested that the extracellular matrix protein thrombospondin-1 (TSP-1) acts as an endogenous inhibitor of corneal lymphangiogenesis via acting on macrophages; ligation of CD36 on the macrophage cell surface by Tsp-1 was shown to negatively regulate VEGF-C and VEGF-D production. In the absence of Tsp-1, and in mice deficient in CD36, precocious lymphangiogenesis is induced in the cornea [70]. In addition to VEGF family members, macrophages are a source of proteases including MMP-2 and MMP-9 [1, 26, 45] that promote growth factor activation and matrix remodelling, as well as cytokines and chemokines that recruit additional cells of the immune system. Immune cells including granulocytes, B and T lymphocytes have also been demonstrated to have pro- and anti lymphangiogenic activities [48, 71–73] and their capacity to regulate lymphangiogenesis should be taken into account when looking at the “big picture” of inflammation.
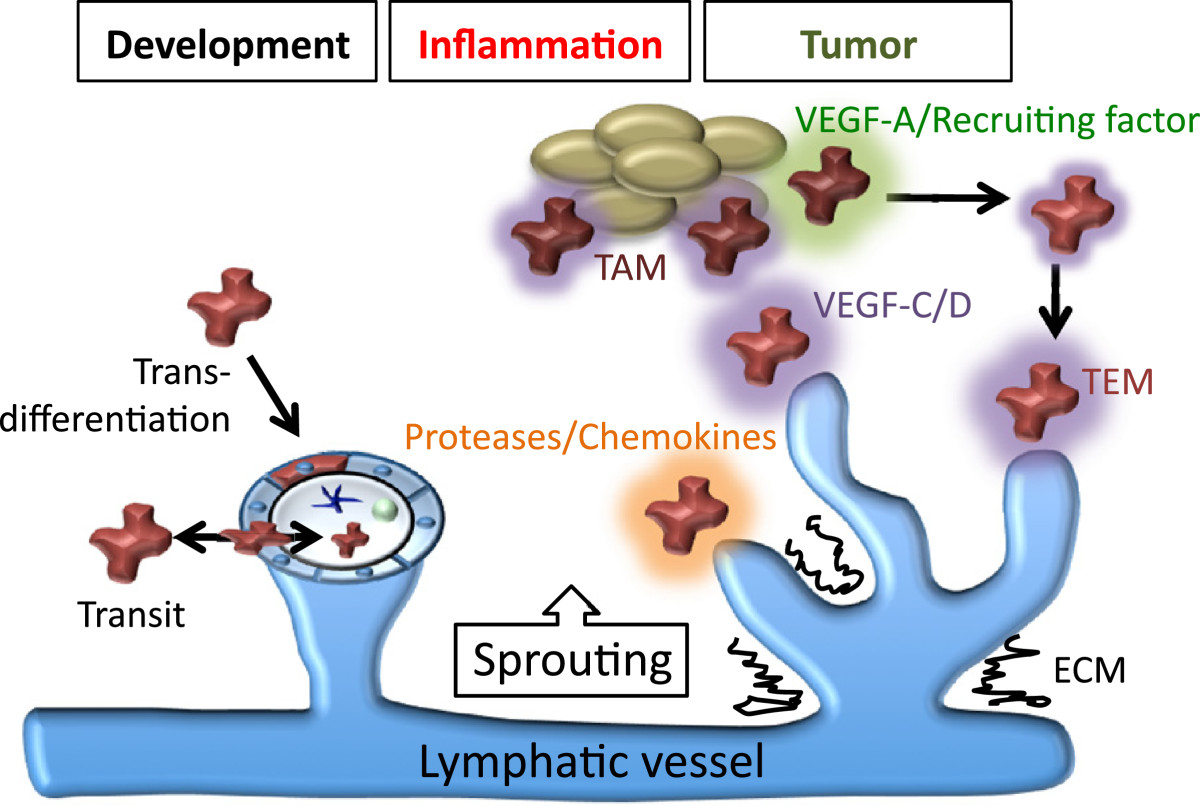
Figure 2
Figure 2 caption
A model depicting the roles of macrophages in lymphangiogenesis. Macrophages have been proposed to contribute to lymphangiogenesis by acting as a source of lymphatic endothelial progenitor cells which incorporate into growing vessels, or by providing growth and patterning signals such as VEGF-A and VEGF-C/D, that stimulate the growth and/or function of the lymphatic vasculature. Lineage tracing studies have suggested that macrophages do not act as lymphatic endothelial progenitor cells, yet they share a close spatial localization with lymphatic vessels during development and express the lymphatic marker LYVE-1. These macrophages may assume localization in the walls of lymphatic vessels in order to perform an immune surveillance role, or be transiting through the lymphatic endothelium. Tumor associated macrophages (TAMs) and Tie2-expressing monocytes (TEMs) promote lymphangiogenesis by liberating proteases important for growth factor activation/matrix remodeling, producing chemokines that degrade the surrounding extracellular matrix (ECM), and recruiting additional inflammatory cells.
Many studies have shown that the growth of lymphatic vessels in the tumor microenvironment facilitates tumor metastasis [74]. Tumor-stimulated lymphangiogenesis often results in the formation of abnormal, leaky lymphatic vessels, a feature that provides metastatic tumor cells with ready access to the lymphatic vasculature [75, 76]. Macrophage recruitment to tumors has been demonstrated to promote lymphangiogenesis in a variety of mouse tumor models [43, 66, 68, 77, 78] and tumor associated macrophages (TAMs) have been linked with increased peri-tumoral lymphangiogenesis and metastasis in human cancers including breast cancer [79], cervical cancer [66], squamous cell carcinoma [80] and advanced colorectal cancer [81]. In a mouse model of osteosarcoma, inhibition of Csf1 diminished macrophage recruitment to the tumor environment, suppressed tumor angiogenesis and lymphangiogenesis and reduced tumor metastasis [43]. Similarly, in a model of urinary bladder cancer, depletion of TAMs with clodronate liposomes and suppression of lymphangiogenesis with soluble VEGFR-3 inhibited lymphangiogenesis and tumor metastasis [77]. Studies such as these suggest that therapeutics designed to block macrophage influx or inhibit macrophage activity might provide valuable anti-tumor and anti-metastatic agents. In addition to promoting the growth of new lymphatic vessels in the vicinity of tumors, macrophage derived pro-lymphangiogenic growth factors including VEGF-C and VEGF-D may act downstream of the initial lymphatics on collecting vessels to promote their dilation and capacity for lymph flow, thereby facilitating metastatic tumor cell transport [35, 82]. TAMs may also contribute to lymphangiogenesis associated pathologies in addition to aiding metastasis. When a metastatic human ovarian cancer cell line was transplanted into mice, Jeon and colleagues found that tumor progression was associated with the development of chylous ascites due to a profound dysfunctional lymphangiogenic response [68]. Blockade of VEGF-C/-D with soluble VEGFR-3 and of VEGF-A signaling with VEGF-Trap prevented the formation of chylous ascites, implicating macrophage derived VEGF-C/-D in ascites development due to the failure of aberrant lymphatic vessels to mediate fluid clearance from the peritoneal cavity [68]. This study has implications for the treatment of ascites in ovarian cancer patients.
Perspectives and future directions
A growing body of data now cements “regulation of lymphangiogenesis during development and disease” together with the plethora of important roles that macrophages play in tissue morphogenesis, homeostasis, repair and immunity. Many questions remain to be answered before we will completely understand how macrophages regulate lymphangiogenesis. Current topical questions include: What is the relative contribution of macrophage derived VEGF-C and -D to embryonic and inflammation stimulated lymphangiogenesis? What signals in addition to VEGF family members do macrophages provide that control lymphatic vascular growth and morphogenesis? Do different macrophage subtypes differ with respect to their pro- or anti- lymphangiogenic activity? The generation of new experimental tools including genetically modified mice and agents able to specifically track and manipulate macrophage sub-types will be important for advancing these studies. Though the blockade of macrophages and/or macrophage derived factors poses an attractive strategy for anti-inflammatory and anti-tumor therapies, it will first be important to determine the precise roles of these intriguing cells in developmental and pathological lymphangiogenesis.
Author contributions
NH and EG wrote the manuscript and prepared the figures. All authors read and approved the final manuscript.
Acknowledgements
NH is supported by project grants from the NHMRC and a fellowship from the National Heart Foundation of Australia. EG is supported by a Yale University School of Medicine Brown-Coxe Fellowship.
Authors’ original submitted files for images
Below are the links to the authors’ original submitted files for images.
Authors’ original file for figure 1
Authors’ original file for figure 2
References
- Trophic macrophages in development and disease. Nat Rev Immunol. 2009;9:259-270.
- The interplay between macrophages and angiogenesis in development, tissue injury and regeneration. Int J Dev Biol. 2011;55:495-503.
- Metchnikoff's policemen: macrophages in development, homeostasis and regeneration. Trends Mol Med. 2011;17:743-752.
- Macrophages are required for cell death and tissue remodeling in the developing mouse eye. Cell. 1993;74:453-462.
- WNT7b mediates macrophage-induced programmed cell death in patterning of the vasculature. Nature. 2005;437:417-421.
- Microglia promote the death of developing Purkinje cells. Neuron. 2004;41:535-547.
- Colony stimulating factor-1 is required to recruit macrophages into the mammary gland to facilitate mammary ductal outgrowth. Dev Biol. 2002;247:11-25.
- Characterisation and trophic functions of murine embryonic macrophages based upon the use of a Csf1r-EGFP transgene reporter. Dev Biol. 2007;308:232-246.
- Dual roles for macrophages in ovarian cycle-associated development and remodelling of the mammary gland epithelium. Development. 2010;137:4229-4238.
- Tissue macrophages act as cellular chaperones for vascular anastomosis downstream of VEGF-mediated endothelial tip cell induction. Blood. 2010;116:829-840.
- Insulin cell mass is altered in Csf1op/Csf1op macrophage-deficient mice. J Leukoc Biol. 2004;76:359-367.
- The osteopetrotic mutation toothless (tl) is a loss-of-function frameshift mutation in the rat Csf1 gene: Evidence of a crucial role for CSF-1 in osteoclastogenesis and endochondral ossification. Proc Natl Acad Sci USA. 2002;99:14303-14308.
- Inflammatory monocytes recruited after skeletal muscle injury switch into antiinflammatory macrophages to support myogenesis. J Exp Med. 2007;204:1057-1069.
- Macrophage Wnt7b is critical for kidney repair and regeneration. Proc Natl Acad Sci USA. 2010;107:4194-4199.
- Selective depletion of macrophages reveals distinct, opposing roles during liver injury and repair. J Clin Invest. 2005;115:56-65.
- A distinguishing gene signature shared by tumor-infiltrating Tie2-expressing monocytes, blood "resident" monocytes, and embryonic macrophages suggests common functions and developmental relationships. Blood. 2009;114:901-914.
- Macrophages define dermal lymphatic vessel calibre during development by regulating lymphatic endothelial cell proliferation. Development. 2010;137:3899-3910.
- Blood monocytes consist of two principal subsets with distinct migratory properties. Immunity. 2003;19:71-82.
- Tie2 identifies a hematopoietic lineage of proangiogenic monocytes required for tumor vessel formation and a mesenchymal population of pericyte progenitors. Cancer Cell. 2005;8:211-226.
- Exploring the full spectrum of macrophage activation. Nat Rev Immunol. 2008;8:958-969.
- Alternative activation of macrophages: mechanism and functions. Immunity. 2010;32:593-604.
- Obligatory participation of macrophages in an angiopoietin 2-mediated cell death switch. Development. 2007;134:4449-4458.
- A two-way communication between microglial cells and angiogenic sprouts regulates angiogenesis in aortic ring cultures. PLoS One. 2011;6:e15846-.
- Regulation of angiogenesis by a non-canonical Wnt-Flt1 pathway in myeloid cells. Nature. 2011;474:511-515.
- VEGF-induced adult neovascularization: recruitment, retention, and role of accessory cells. Cell. 2006;124:175-189.
- Vascular-leukocyte interactions: mechanisms of human decidual spiral artery remodeling in vitro. Am J Pathol. 2010;177:1017-1030.
- Contribution of monocytes/macrophages to compensatory neovascularization: the drilling of metalloelastase-positive tunnels in ischemic myocardium. Circ Res. 2000;87:378-384.
- Three pathways to mature macrophages in the early mouse yolk sac. Blood. 2005;106:3004-3011.
- Fate mapping analysis reveals that adult microglia derive from primitive macrophages. Science. 2010;330:841-845.
- Prox1 function is required for the development of the murine lymphatic system. Cell. 1999;98:769-778.
- Segmental territories along the cardinal veins generate lymph sacs via a ballooning mechanism during embryonic lymphangiogenesis in mice. Dev Biol. 2012;364:89-98.
- Vascular endothelial growth factor C is required for sprouting of the first lymphatic vessels from embryonic veins. Nat Immunol. 2004;5:74-80.
- Sox18 induces development of the lymphatic vasculature in mice. Nature. 2008;456:643-647.
- The nuclear hormone receptor Coup-TFII is required for the initiation and early maintenance of Prox1 expression in lymphatic endothelial cells. Genes Dev. 2010;24:696-707.
- Lymphangiogenesis: Molecular mechanisms and future promise. Cell. 2010;140:460-476.
- Current views on the function of the lymphatic vasculature in health and disease. Genes Dev. 2010;24:2115-2126.
- A genetic Xenopus laevis tadpole model to study lymphangiogenesis. Nat Med. 2005;11:998-1004.
- Mesenchymal cells with leukocyte and lymphendothelial characteristics in murine embryos. Dev Dyn. 2006;235:1554-1562.
- Dual origin of avian lymphatics. Dev Biol. 2006;292:165-173.
- Proliferating mesodermal cells in murine embryos exhibiting macrophage and lymphendothelial characteristics. BMC Dev Biol. 2008;8:43-.
- Lineage tracing demonstrates the venous origin of the mammalian lymphatic vasculature. Genes Dev. 2007;21:2422-2432.
- Identification of proangiogenic TIE2-expressing monocytes (TEMs) in human peripheral blood and cancer. Blood. 2007;109:5276-5285.
- M-CSF inhibition selectively targets pathological angiogenesis and lymphangiogenesis. J Exp Med. 2009;206:1089-1102.
- Total absence of colony-stimulating factor 1 in the macrophage-deficient osteopetrotic (op/op) mouse. Proc Natl Acad Sci USA. 1990;87:4828-4832.
- Regulation of developmental lymphangiogenesis by Syk(+) leukocytes. Dev Cell. 2010;18:437-449.
- The development of functional B lymphocytes in conditional PU.1 knock-out mice. Blood. 2005;106:2083-2090.
- Conditional deletion of the colony stimulating factor-1 receptor (c-fms proto-oncogene) in mice. Genesis. 2006;44:328-335.
- Pathogenesis of persistent lymphatic vessel hyperplasia in chronic airway inflammation. J Clin Invest. 2005;115:247-257.
- Critical role of CD11b + macrophages and VEGF in inflammatory lymphangiogenesis, antigen clearance, and inflammation resolution. Blood. 2009;113:5650-5659.
- An important role of lymphatic vessel activation in limiting acute inflammation. Blood. 2011;117:4667-4678.
- Lymphatic neoangiogenesis in human kidney transplants is associated with immunologically active lymphocytic infiltrates. J Am Soc Nephrol. 2004;15:603-612.
- Targeting lymphangiogenesis after islet transplantation prolongs islet allograft survival. Transplantation. 2011;92:25-30.
- Clinicopathological correlation analysis of (lymph) angiogenesis and corneal graft rejection. Mol Vis. 2011;17:1694-1700.
- Role of CD11b + macrophages in intraperitoneal lipopolysaccharide-induced aberrant lymphangiogenesis and lymphatic function in the diaphragm. Am J Pathol. 2009;175:1733-1745.
- Inflammation-induced lymphangiogenesis in the cornea arises from CD11b-positive macrophages. J Clin Invest. 2005;115:2363-2372.
- Decreased macrophage number and activation lead to reduced lymphatic vessel formation and contribute to impaired diabetic wound healing. Am J Pathol. 2007;170:1178-1191.
- Lymphatic endothelial progenitor cells contribute to de novo lymphangiogenesis in human renal transplants. Nat Med. 2006;12:230-234.
- The maintenance of lymphatic vessels in the cornea is dependent on the presence of macrophages. Invest Ophthalmol Vis Sci. 2012;53:3145-3153.
- Increased lymphangiogenesis in joints of mice with inflammatory arthritis. Arthritis Res Ther. 2007;9:R118-.
- Lymphangiogenesis is required for pancreatic islet inflammation and diabetes. PLoS One. 2011;6:e28023-.
- Shi VY, Bao L, Chan LS: Inflammation-driven Dermal Lymphangiogenesis in Atopic Dermatitis is Associated with CD11b+ Macrophage Recruitment and VEGF-C Up-regulation in the IL-4-Transgenic Mouse Model. Microcirculation. in press
- Macrophages regulate salt-dependent volume and blood pressure by a vascular endothelial growth factor-C-dependent buffering mechanism. Nat Med. 2009;15:545-552.
- Podoplanin-expressing cells derived from bone marrow play a crucial role in postnatal lymphatic neovascularization. Circulation. 2010;122:1413-1425.
- New model of macrophage acquisition of the lymphatic endothelial phenotype. PLoS One. 2012;7:e31794-.
- Myeloid cells contribute to tumor lymphangiogenesis. PLoS One. 2009;4:e7067-.
- Tumor-associated macrophages express lymphatic endothelial growth factors and are related to peritumoral lymphangiogenesis. Am J Pathol. 2002;161:947-956.
- VEGF-A stimulates lymphangiogenesis and hemangiogenesis in inflammatory neovascularization via macrophage recruitment. J Clin Invest. 2004;113:1040-1050.
- Profound but dysfunctional lymphangiogenesis via vascular endothelial growth factor ligands from CD11b + macrophages in advanced ovarian cancer. Cancer Res. 2008;68:1100-1109.
- Induction of cutaneous delayed-type hypersensitivity reactions in VEGF-A transgenic mice results in chronic skin inflammation associated with persistent lymphatic hyperplasia. Blood. 2004;104:1048-1057.
- Thrombospondin 1 inhibits inflammatory lymphangiogenesis by CD36 ligation on monocytes. J Exp Med. 2011;208:1083-1092.
- B lymphocyte-specific c-Myc expression stimulates early and functional expansion of the vasculature and lymphatics during lymphomagenesis. Am J Pathol. 2003;163:2233-2245.
- B cell-driven lymphangiogenesis in inflamed lymph nodes enhances dendritic cell mobilization. Immunity. 2006;24:203-215.
- T lymphocytes negatively regulate lymph node lymphatic vessel formation. Immunity. 2011;34:96-107.
- Focus on lymphangiogenesis in tumor metastasis. Cancer Cell. 2005;7:121-127.
- Distinct architecture of lymphatic vessels induced by chimeric vascular endothelial growth factor-C/vascular endothelial growth factor heparin-binding domain fusion proteins. Circ Res. 2007;100:1468-1475.
- Vascular endothelial cell growth factor receptor 3-mediated activation of lymphatic endothelium is crucial for tumor cell entry and spread via lymphatic vessels. Cancer Res. 2005;65:4739-4746.
- Soluble vascular endothelial growth factor receptor-3 suppresses lymphangiogenesis and lymphatic metastasis in bladder cancer. Mol Cancer. 2011;10:36-.
- Alternatively activated RAW264.7 macrophages enhance tumor lymphangiogenesis in mouse lung adenocarcinoma. J Cell Biochem. 2009;107:134-143.
- VEGF-C expressing tumor-associated macrophages in lymph node positive breast cancer: impact on lymphangiogenesis and survival. Surgery. 2006;139:839-846.
- The human cutaneous squamous cell carcinoma microenvironment is characterized by increased lymphatic density and enhanced expression of macrophage-derived VEGF-C. J Invest Dermatol. 2011;131:229-236.
- Type and location of tumor-infiltrating macrophages and lymphatic vessels predict survival of colorectal cancer patients. Int J Cancer. 2011;131:864-873.
- VEGF-D promotes tumor metastasis by regulating prostaglandins produced by the collecting lymphatic endothelium. Cancer Cell. 2012;21:181-195.