Notch4 is required for tumor onset and perfusion
Vascular Cell. 2013;
Received: 19 February 2013 | Accepted: 5 April 2013 | Published: 20 April 2013
Vascular Cell ISSN: 2045-824X
Abstract
Background
Notch4 is a member of the Notch family of receptors that is primarily expressed in the vascular endothelial cells. Genetic deletion of
Methods
We examined the requirement for Notch4 in the development of breast cancer vasculature. Orthotopic transplantation of mouse mammary tumor cells wild type for
Results
Here, we show that Notch4 expression is required for tumor onset and early tumor perfusion in a mouse model of breast cancer. We found that Notch4 expression is upregulated in mouse and human mammary tumor vasculature. Moreover, host
Conclusion
Our results suggest a novel role for Notch4 in the establishment of tumor colonies and vessel perfusion of transplanted mammary tumors.
Keywords
Notch Angiogenesis Tumor Blood vessel PerfusionBackground
Notch signaling underlies an evolutionarily conserved mechanism that regulates a wide range of cellular processes via direct cell-cell communication. In mammals, there are five Notch ligands (Jagged1, Jagged2, Delta-like 1/Dll1, Dll3, and Dll4) and four Notch receptors (Notch1-4). The interaction between the Notch ligand and its receptor triggers two proteolytic cleavages that release the Notch intracellular domain into the nucleus. There, the Notch intracellular domain forms complexes with members of the CSL transcription factor family (CBF1/RBP-Jκ/Suppressor of Hairless/LAG-1), leading to the expression of downstream transcription factors (reviewed in [1]).
Among the four Notch receptors, Notch1 and Notch4 are expressed in the vascular endothelium, with Notch4 expression being more restricted to endothelial cells (ECs) [2]. Mice homozygous for a null allele of
Angiogenesis, the formation of new vessels from pre-existing ones, plays a key role in cancer and other pathological conditions. Solid tumors depend on the development of blood vessels to provide oxygen and nutrients to support their growth [6]. Interfering with Notch1 signaling dramatically affects angiogenesis in the developing and pathological vasculatures (reviewed in [7, 8]). In solid tumors, blocking Dll4 leads to excessive sprouting and “non-productive” angiogenesis, which results in decreased vessel perfusion and inhibition of tumor growth [9, 10]. Additionally, selective blocking of Notch1 with antibodies inhibits tumor growth by a mechanism consistent with that of Dll4 inhibition, namely by promoting abnormal growth of vessel sprouts that do not perfuse tissues [11]. Thus while the importance of the Dll4-Notch1 signaling axis in tumor angiogenesis is well documented, whether Notch4, primarily expressed in the endothelium, is required for angiogenesis, vascular perfusion, and tumor progression is unknown.
To unravel the role of Notch4 in tumor angiogenesis, we chose breast cancer as a solid tumor model. Since Notch4 is predominantly expressed in the vascular endothelium in mice [2], we therefore used orthotopic transplantation of mouse mammary carcinoma cells wild type for
Methods
Animals and human samples
C57BL/6J wild type mice were purchased from Jackson Laboratory
Human breast cancer samples (paraffin-embedded tissue blocks) were obtained from the UCSF Breast Cancer SPORE tissue core, which collected samples in compliance with a protocol approved by the UCSF Committee on Human Research. Patient consent was obtained using the standard surgical consent form, in combination with the brochure, Donating Tissue for Medical Research. The research involved collection of left-over specimens and, as such, involved no more than minimal risk to the subjects. Our use of these tissues was also approved by the UCSF Committee on Human Research.
Immunostaining
Paraffin tissue sections of human samples were processed using standard methods. Mouse tissue samples were fixed in 4% formaldehyde in phosphate-buffered saline (PBS), embedded in OCT (Tissue Tek), frozen, and cryosectioned. Immunostaining was performed using rabbit anti-Notch4 (Upstate, diluted 1/1000) and rat anti-mouse CD31 (MEC 13.3, Pharmingen, diluted 1/500) as previously described [12, 13]. Secondary antibodies were Cy3-conjugated donkey anti-rabbit (diluted 1/1000) and alexa-488 donkey anti-rat (diluted 1/1000), both from Jackson ImmunoResearch. In experiments with mouse tissue tumors, sections were collected from three different regions of each tumor and quantification was performed from three different fields per section. When tumors were not detected by naked eye, the whole mammary fat pad was preserved for sectioning and microscopical analysis.
Animal procedures
Animal experiments were performed in compliance with the guidelines of UCSF Institutional Animal Care and Use Committee (IACUC), who approved this study under protocol #AN085404 and AN075264. We routinely monitored our mice targeted for tumorigenesis before and during tumor formation. Mice that displayed any of the following signs were euthanized: loss of 15% of their initial body weight, tumor size of 2 cm in the largest dimension, or decline in activity.
Isolation of primary tumor cells and orthotopic transplantation
Primary tumor epithelial cells were isolated from mammary carcinomas that developed in
Vessel perfusion studies
Mice were anesthetized with isoflurane and injected in the tail vein with a combination of 60 μg of biotinylated
Real-time PCR
Total RNA was isolated from normal mammary inguinal fat pads and from transplanted tumors using Trizol (Invitrogen), following the manufacturer’s instructions. RNA was further purified from contaminant genomic DNA with DNase I treatment and RNeasy columns (Qiagen), following the manufacturer’s instructions. RNA was retro-transcribed using SuperscriptIII (Invitrogen) and SYBR Green-based real-time-PCR was used to analyze gene expression. Normalized fold change in gene expression in tumor relative to normal gland was calculated according to Pffafl [15], using
Immunoprecipitation and western blotting
Immunoprecipitation of Notch4 from lysates of normal mammary gland at pubertal stage followed by Western blotting analysis was performed as previously described [16] using a polyclonal rabbit anti-Notch4 antibody (Upstate).
Statistical analysis
Vessel density and perfusion analysis was performed as previously described [13]. Data are expressed as mean + standard error of the mean (s.e.m). P-values were calculated using a two-tailed t test except for tumor onset analysis where Fisher’s exact test was applied. Values of p≤0.05 were considered statistically significant.
Results
Notch4 expression is upregulated in the vasculature of mouse and human mammary tumors
To test the hypothesis that Notch4 is involved in pathological angiogenesis, we used mammary tumors as a model. We first assessed whether an orthotopic tumor model derived from the
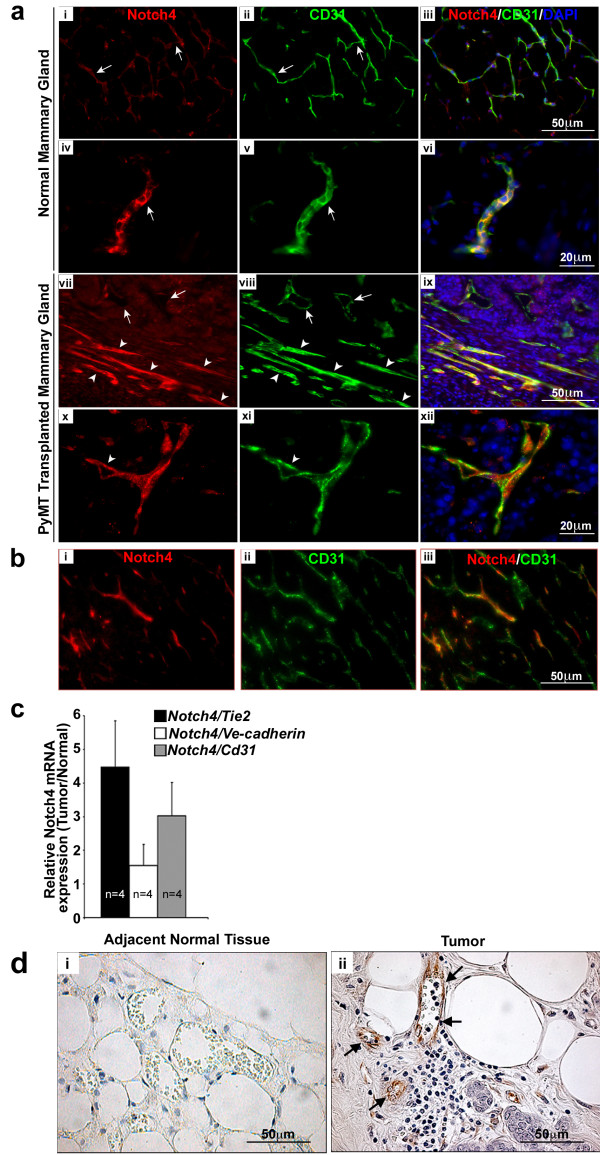
Figure 1
Figure 1 caption
Notch4 expression is upregulated in the vasculature of mouse and human mammary tumors. (a) Notch4/CD31 immunostaining reveals an increase of Notch4 expression in the vasculature of mouse orthotopic mammary tumors (vii-xii) compared to age and stage matched (virgin 8 weeks old) normal mouse mammary glands (i-vi). In panels vii-viii arrows indicate Notch4 low-expressing vessels; arrowheads indicate Notch4 positive vessels. Panels i to iii show low expression levels of Notch4 and CD31 in the vessels of normal mammary gland. Panels iv to vi show a detail of the staining of Notch4 and CD31 in the vessels of normal mammary gland. Panels vii-xii show Notch4 and CD31 staining in tumors without adjacent normal tissues in these images. Panels vii and ix show higher expression of Notch4 in vessels of the tumors than those in the normal gland (as compared to panels i and iii). Panels x (Notch4 staining), xi (CD31 staining) and xii (merged) show higher magnification of tumor vessels with Notch4 expression. (b) Notch4 staining in the tumor is mainly restricted to the vasculature. Orthotropic mammary tumors at 3 weeks post transplant were immunostained for (i) Notch4, and (ii) CD31. Panel iii shows the merge of Notch4 and CD31. (c) mRNA expression is increased in orthotopic tumors derived from transplanted cells compared to normal mammary tissue as measured by quantitative RT-PCR. Data is represented as fold change of expression in tumor over normal mammary glands, normalized to the expression of , and . Samples were analyzed in duplicate and error bars represent standard error of mean (s.e.m). (d) Notch4 immunostaining (brown stain) is increased in the vasculature of human infiltrating ductal carcinoma tissue (ii) compared to adjacent normal tissue (i). Arrows indicate Notch4 staining in the endothelium.
To corroborate our immunostaining data,
Next, we examined human biopsy samples (n=6) from infiltrating ductal carcinoma, which were immunostained with an antibody against Notch4. We found increased Notch4 expression specifically in the tumor vasculature (Figure 1d, ii) relative to blood vessels in the adjacent normal tissue (Figure 1d, i). This result indicates that, consistent with the finding in mice, up-regulation of vascular Notch4 also occurs in human breast tumorigenesis and suggests that host Notch4 may play a role in breast tumor angiogenesis.
Taken together, our results show that Notch4 expression is upregulated in the tumor vasculature in both murine and human mammary tumors, suggesting a possible role for this receptor in breast cancer formation and angiogenesis.
Tumor onset is delayed in the absence of host Notch4, along with poor vessel perfusion
To study the function of Notch4 during angiogenesis in mammary tumors, we took advantage of the orthotopic tumor model described above. This model lets us test host Notch4 effects independently of tumor Notch4 effects, because tumor cells from a
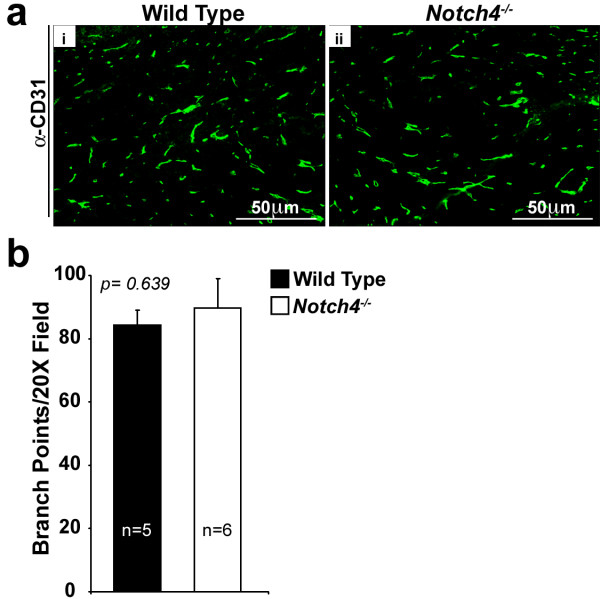
Figure 2
Figure 2 caption
Vessel density is comparable in-deficient and wild type mouse mammary glands. (a) CD31 immunostaining (green) pattern is similar in wild type (i) and (ii) mouse mammary glands. (b) Vessel density (represented as branch points/20X field in intact inguinal mammary fat pads) is similar in wild type and mice. Bars represent average values and error bars represent s.e.m.
We next compared the onset of palpable tumors in wild type and
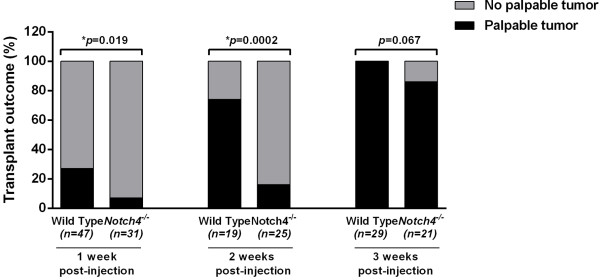
Figure 3
Figure 3 caption
Host Notch4 deficiency delays onset of tumors derived from orthotopic transplantation ofcells. Incidence of palpable tumors in wild type and mice at 1, 2 and 3 weeks post orthotopic transplantation of tumor cells. Tumor onset is significantly delayed in mice at the early time points.
Given that Notch4 is predominantly expressed in the normal endothelium and upregulated in the tumor vasculature, we examined blood vessels of tumors transplanted into wild type and
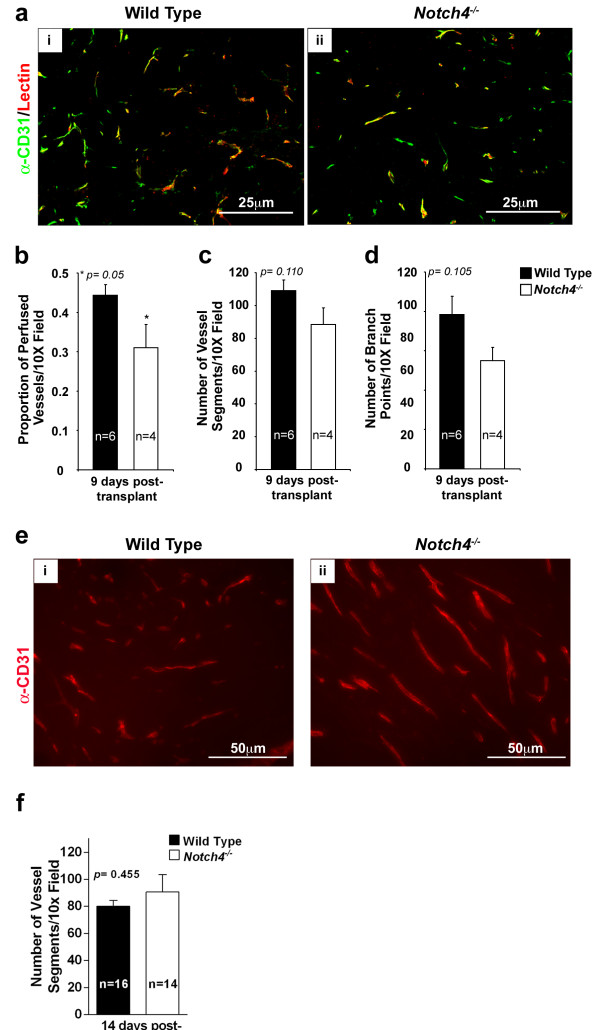
Figure 4
Figure 4 caption
Vascular perfusion is compromised in tumors developed inhosts whereas vessel density is unaffected. (a) CD31 immunostaining (green) and lectin-perfused (red) blood vessel patterns in orthotopic tumors are similar in wild type (i) and (ii) hosts. (b) Vessel perfusion in tumors is decreased in hosts compared to wild type hosts 9 days after orthotopic transplantation. (c and d) Vessel density, either measured as number of vessel segments (c) or as number of branch points (d) in tumors is similar in wild type and hosts 9 days after orthotopic transplantation. (e) CD31 staining in tumors developed in wild type (i) and (ii) hosts 2 weeks after transplantation. (f) Vessel density in tumors is similar in wild type and hosts 2 weeks after orthotopic transplantation. Bars represent average values and error bars represent s.e.m.
Next we examined whether host Notch4 was required for tumor progression after tumor onset. Tumor-bearing wild type and
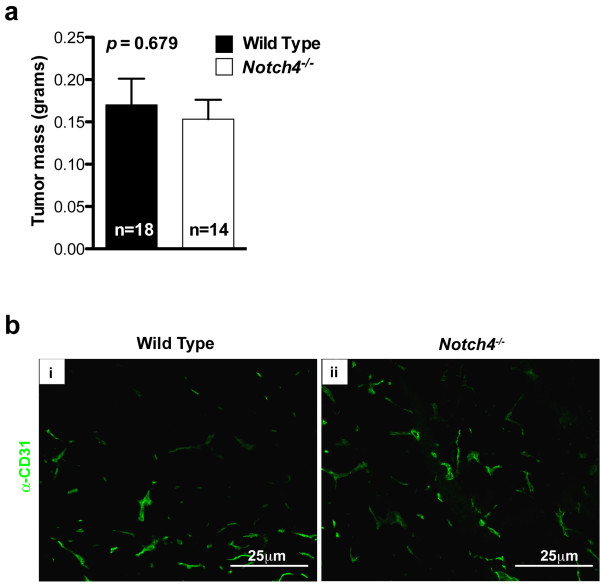
Figure 5
Figure 5 caption
Tumor mass and vascular architecture are similar in tumors developed in wild type andhosts at later stages. (a) Tumor mass of tumors at 3 weeks after orthotopic transplantation. Bars represent average values and error bars represent s.e.m. (b) CD31 staining highlighting tumor vascular architecture 3 weeks after orthotopic transplantation.
Discussion
In this study we demonstrate that Notch4 expression is upregulated in the vasculature of mammary tumors. Furthermore, our results suggest that host Notch4 plays a role in the early emergence of
Our results show increased levels of Notch4 in the blood vessels of mouse and human breast tumor tissues. Expression of Notch receptors and ligands has been described in a wide variety of different tumor types, such as colorectal, prostate, liver, pancreatic and breast cancer [23, 24] and a role for Notch4 in regulating breast cancer stem cell activity has been proposed [25]. Mittal
Orthotopic transplantation of mammary tumor cells is a well-established model for
Although poor tumor vessel perfusion correlated significantly with delayed tumor onset at early time points after transplantation, our results alone do not provide causal proof that reduced vessel perfusion leads to delayed tumor onset in
Host Notch4 deficiency delays tumor onset and decreases initial perfusion, however, the growth of established tumors can ultimately progress in the absence of host Notch4. This result suggests that host Notch4 plays a unique role in the initiation of tumor onset after transplantation.
Surprisingly, our results indicate that Notch4 is dispensable for vessel sprouting in the tumor. Sprouting angiogenesis is a hallmark of tumor neovascularization, and Dll4/Notch1 signaling functions to inhibit vessel sprouting [8]. Moreover, given that Notch1 appears to be the primary Notch receptor responsible for developmental angiogenesis [3], together with the results obtained using specific anti-Notch1 antibodies in tumors [11], Notch1 seems to be the predominant mediator of Notch signaling in tumor angiogenesis. It is therefore likely that the increased vascular network observed when inhibiting pan-Notch signaling or Notch ligands is mainly due to the inhibition of Notch1. Alternatively, it is possible that a subtle defect in vessel sprouting exists in the
Conclusion
Our finding that the lack of
Availability of supporting data
The data set supporting the results of this article is included within the article (and its Additional file 1: Figure S1).
Additional files
Additional file 1: Figure S1: Mammary glads from Notch4-/-mice exhibit no detectable Notch4 protein expression. Notch4 immunoprecipitation-immunoblot analysis on mammary tissue lysates from wild type (lane 1), Notch+/- (lane 3), and Notch4-/- (lane 5) mice shows a lack of Notch4 protein in Notch4-/- mice. Lanes 2, 4 and 6 are the same samples immunoprecipiated with a control rabbit lαG. (PDF 463 KB)
Acknowledgments
We thank the laboratories of Drs. J. Michael Bishop and Zena Werb for advice with the
Authors’ original submitted files for images
Below are the links to the authors’ original submitted files for images.
Authors’ original file for figure 1
Authors’ original file for figure 2
Authors’ original file for figure 3
Authors’ original file for figure 4
Authors’ original file for figure 5
References
- Notch signaling: the core pathway and its posttranslational regulation. Dev Cell. 2009;16(5):633-647.
- Notch4/int-3, a mammary proto-oncogene, is an endothelial cell-specific mammalian Notch gene. Development. 1996;122(7):2251-2259.
- Notch signaling is essential for vascular morphogenesis in mice. Genes Dev. 2000;14(11):1343-1352.
- Notch1 is essential for postimplantation development in mice. Genes Dev. 1994;8(6):707-719.
- Critical role of endothelial Notch1 signaling in postnatal angiogenesis. Circ Res. 2007;100(1):70-78.
- Tumor angiogenesis: therapeutic implications. N Engl J Med. 1971;285(21):1182-1186.
- Notch signaling in developmental and tumor angiogenesis. Genes Cancer. 2011;2(12):1106-1116.
- Angiogenesis: a team effort coordinated by notch. Dev Cell. 2009;16(2):196-208.
- Blockade of Dll4 inhibits tumour growth by promoting non-productive angiogenesis. Nature. 2006;444(7122):1032-1037.
- Inhibition of Dll4 signalling inhibits tumour growth by deregulating angiogenesis. Nature. 2006;444(7122):1083-1087.
- Therapeutic antibody targeting of individual Notch receptors. Nature. 2010;464(7291):1052-1057.
- Endothelial FAK is essential for vascular network stability, cell survival, and lamellipodial formation. J Cell Biol. 2006;172(1):151-162.
- Endothelial Notch signaling is upregulated in human brain arteriovenous malformations and a mouse model of the disease. Lab Invest. 2009;89(9):971-982.
- MET and MYC cooperate in mammary tumorigenesis. Proc Natl Acad Sci USA. 2005;102(12):4324-4329.
- A new mathematical model for relative quantification in real-time RT-PCR. Nucleic Acids Res. 2001;29(9):e45-.
- Activation of the Met receptor by cell attachment induces and sustains hepatocellular carcinomas in transgenic mice. J Cell Biol. 2001;153(5):1023-1034.
- Identification of conserved gene expression features between murine mammary carcinoma models and human breast tumors. Genome Biol. 2007;8(5):R76-.
- Induction of mammary tumors by expression of polyomavirus middle T oncogene: a transgenic mouse model for metastatic disease. Mol Cell Biol. 1992;12(3):954-961.
- Vascular remodelling during the normal and malignant life cycle of the mammary gland. Microsc Res Tech. 2001;52(2):182-189.
- Inactivation of VEGF in mammary gland epithelium severely compromises mammary gland development and function. FASEB J. 2007;21(14):3994-4004.
- Expression of notch receptors, notch ligands, and fringe genes in hematopoiesis. Exp Hematol. 2000;28(5):527-534.
- Angiogenesis: a marker for neoplastic transformation of mammary papillary hyperplasia. Science. 1977;195(4281):880-882.
- Recent insights into the role of Notch signaling in tumorigenesis. Blood. 2006;107(6):2223-2233.
- Notch signalling in solid tumours: a little bit of everything but not all the time. Nat Rev Cancer. 2011;11(5):338-351.
- Regulation of breast cancer stem cell activity by signaling through the Notch4 receptor. Cancer Res. 2010;70(2):709-718.
- Cooperation of Notch and Ras/MAPK signaling pathways in human breast carcinogenesis. Mol Cancer. 2009;8:128-.
- Notch-1 and Notch-4 biomarker expression in triple-negative breast cancer. Int J Surg Pathol. 2012;20(2):139-145.
- Notch4 intracellular domain binding to Smad3 and inhibition of the TGF-beta signaling. Oncogene. 2005;24(34):5365-5374.
- Expression of vascular notch ligand delta-like 4 and inflammatory markers in breast cancer. Am J Pathol. 2010;176(4):2019-2028.
- Basic and therapeutic aspects of angiogenesis. Cell. 2011;146(6):873-887.
- Inhibition of Dll4-mediated signaling induces proliferation of immature vessels and results in poor tissue perfusion. Blood. 2007;109(11):4753-4760.
- Accessories to the crime: functions of cells recruited to the tumor microenvironment. Cancer Cell. 2012;21(3):309-322.