Effects of a novel cyclic RGD peptidomimetic on cell proliferation, migration and angiogenic activity in human endothelial cells
Vascular Cell. 2014;
Received: 28 October 2013 | Accepted: 17 April 2014 | Published: 21 May 2014
Vascular Cell ISSN: 2045-824X
Abstract
Background
Cyclic RGD peptidomimetics containing a bifunctional diketopiperazine scaffold are a novel class of high-affinity ligands for the integrins αVβ3 and αVβ5. Since integrins are a promising target for the modulation of normal and pathological angiogenesis, the present study aimed at characterizing the ability of the RGD peptidomimetic
Methods
Cell viability was assessed by flow cytometry and annexin V (ANX)/propidium iodide (PI) staining. Cell proliferation was evaluated by the ELISA measurement of bromodeoxyuridine (BrdU) incorporation. Network formation by HUVEC cultured in Matrigel-coated plates was evaluated by optical microscopy and image analysis. Integrin subunit mRNA expression was assessed by real time-PCR and Akt phosphorylation by western blot analysis.
Results
Conclusions
Keywords
RGD peptidomimetics Integrins Angiogenesis Human umbilical vein endothelial cells Interleukin-8Introduction
Angiogenesis, the growth of new blood vessels as sprouts or offshoots of the pre-existing microvasculature, is a physiological event occurring in the development of organisms, wound healing and the reproductive cycle, but it is also involved in pathologic processes such as inflammation, tumour growth and metastasis [1]. Angiogenesis can be stimulated by a large number of pro-angiogenic cytokines, such as vascular endothelial growth factor (VEGF), tumour necrosis factor α (TNF-α), basic fibroblast growth factor (bFGF) and interleukin-8 (IL-8) [2, 3].
Among the proteins involved in the angiogenic process, integrins play an important role by promoting endothelial cell attachment and migration on the surrounding extracellular matrix, cell to cell interaction and intracellular signal transduction [4]. Integrins are heterodimeric proteins composed of two non covalently associated α and β transmembrane glycoproteins; 18 α and 8 β subunits that give rise to 24 possible distinct integrin proteins [5, 6]. Across their extracellular α/β subunit interface containing the metal ion-dependent adhesion site (MIDAS), integrins recognize and bind protein ligands through contiguous tripeptide sequences, the majority of which are present within flexible loop regions and contain an acidic residue [7]. Several integrins, including αV, α5β1 and αIIbβ3 integrins, recognize the Arg-Gly-Asp (RGD) sequence in endogenous ligands. The context of the ligand RGD sequence (flanking residues, three dimensional presentation) and individual features of the integrin binding pockets determine the recognition specificity and efficacy. These observations prompted many research groups to investigate the use of conformationally constrained cyclic RGD peptides and peptidomimetics as active and selective integrin ligands [8, 9]. One of these, Cilengitide, namely cyclo-[Arg-Gly-Asp-D-Phe-N(Me)-Val] is currently in phase III clinical trials as an angiogenesis inhibitor for patients with glioblastoma multiforme alone [10] or in combination with other antiblastic drugs [11]. Recently, RGD compounds have been proposed also as targeting ligands for integrins in order to better characterize tumor neovascularisation [12]. Notwithstanding these results, the mechanism of RGD ligands in the inhibition of angiogenesis is not yet fully understood, as significant cross-talk exists in the regulation of angiogenesis between integrin operated pathways and, for instance, VEGF receptor pathways [13], and on these bases it has been proposed that agents able to inhibit multiple pathways would have important therapeutic potential [14].
Recently, some of us reported a new class of cyclic RGD peptidomimetics containing a bifunctional diketopiperazine (DKP) scaffold, showing a low nanomolar affinity for integrins αVβ3 and αVβ5[15, 16]. The present study is aimed at characterizing the ability of the cyclic RGD peptidomimetic
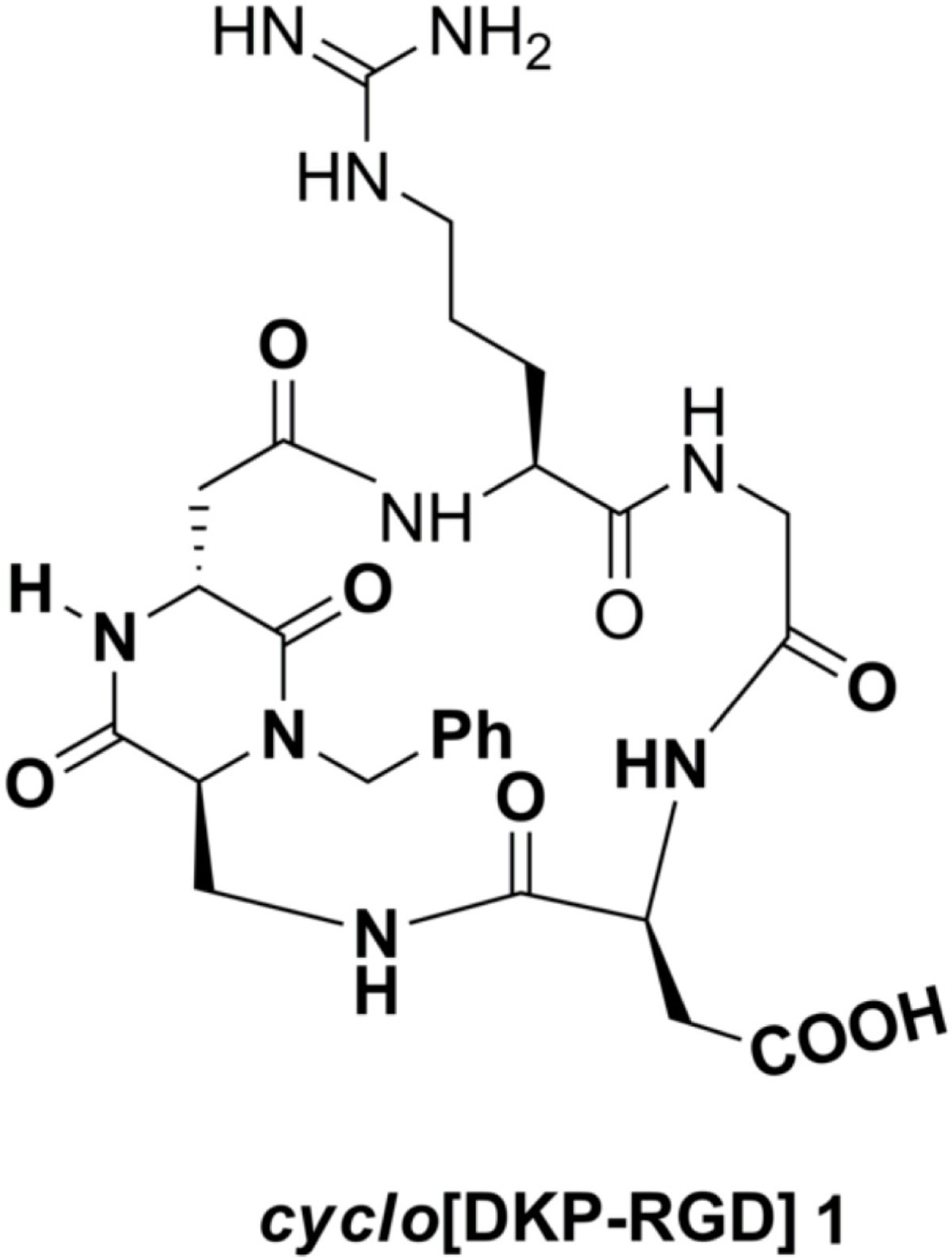
Figure 1
Figure 1 caption
Structure of the peptidomimetic[DKP-RGD] 1.
Materials and methods
Reagents
The peptidomimetic
Cell cultures
HUVEC were cultured in a medium supplemented with FBS (2%), L-glutamine (10 mM), heparin sulphate (0.75 U/ml), VEGF (5 ng/ml), EGF (5 ng/ml), FGF2 (5 ng/ml), IGF-I (15 ng/ml) and ascorbic acid (50 μg/ml) at 37°C, in a moist atmosphere of 5% CO2. HUVEC were used for the experiments between passage 2 and 8. All the experiments were conducted under two different conditions: basal conditions (resting) i.e. cell cultured in EndoGRO basal medium alone, and stimulated conditions, i.e. with the addition of VEGF (5 ng/ml), EGF (5 ng/ml), FGF2 (5 ng/ml), IGF-I (15 ng/ml) together with 10% FBS. In viability, proliferation and migration assays, cells were used after overnight culture in EndoGRO basal medium alone (starvation).
Cell viability
Cell viability assay was performed by flow cytometry. Briefly, after treatment HUVEC were detached with a trypsin solution, centrifuged at 600 g for 5 min at room temperature and the supernatant was finally removed. The cell pellet was resuspended in 100 μL Binding Buffer 1× with the addition of 5 μL annexin V (ANX)-FITC and 5 μL PI, and finally incubated for 15 min at room temperature in the dark. Samples were stored on ice and analyzed without washing. Acquisition was performed on a BD FACSCanto II flow cytometer (Becton Dickinson Italy, Milan, Italy) and data were analyzed using BD FACSDiva software (version 6.1.3). HUVEC were identified on the basis of forward-scatter (FSC) and side-scatter (SSC) properties, and a minimum of 15000 cells for each sample was collected in the gate. Viable, apoptotic and necrotic HUVEC were identified on a biparametric plot ANX-FITC vs PI. Data were finally expressed as% viable (ANX-/PI-), early apoptotic cells (ANX+/PI-), late apoptotic/necrotic cells (ANX+/PI+) and necrotic cells (ANX-/PI+).
Proliferation assay
To assess HUVEC proliferation, 1×104 cells were seeded in duplicate in a 96-well plate and cultured for 24 h without or with
Cell migration assay
Cell migration was measured by means of a Boyden chamber assay. Briefly, 1×105 HUVEC were seeded in the top well of the Boyden chamber,
Angiogenesis assay
To assess angiogenic activity, HUVEC 2.5×104 cells were seeded in a 24-well plate coated with 100 μl/well of Matrigel previously polymerized for 1 h at 37°C. Cells were then incubated for 5 h at 37°C in a moist atmosphere of 5% CO2 without or with
Real time PCR
Cells were treated for 5 h in the presence or absence of 1 μM cyclo[DKP-RGD] 1 in different growth conditions, as previously described. At the end of the treatment, RNA extraction was performed using the Qiazol lysis reagent. Primers were designed by using the “Primer3 input” software (http://frodo.wi.mit.edu/cgi-bin/primer3/primer3.cgi/primer3_www.cgi) and the specificity of each primer was controlled by the BLAST software (http://blast.ncbi.nlm.nih.gov) (Table 1). Real time PCR was performed as previously reported [18] At the end of the reaction, a melting curve analysis was carried out to check for the presence of primer-dimers. Comparison of the expression of each gene was determined by using GAPDH as housekeeping gene. Each run was analyzed in duplicate and data are finally expressed as 2-Δct.
Table 1
Gene | Ref. sequence | Sequence | Product size |
---|---|---|---|
αv | NM_002210 | Forward: actggcttaagagagggctgtg | 110 |
Reverse: tgccttacaaaaatcgctga | |||
β3 | NM_000212 | Forward: agacactcccacttggcatc | 123 |
Reverse: tcctcaggaaaggtccaatg | |||
β5 | NM_002213 | Forward: agcctatctccacgcacact | 91 |
Reverse: cctcggagaaggaaacatca | |||
GAPDH | NM_001289746.1 | Forward: caactgtgaggaggggagatt | 97 |
Reverse: cagcaagagcacaagaggaag |
Western blot analysis
Cells grown in 60-mm dishes were treated for 5 h with 1 μM
Statistical analysis
Data are shown as means ± standard deviation (SD) unless otherwise indicated. Statistical significance of the differences was assessed by two-tailed Student’s
Results
Viability and apoptosis
Viable cells, measured after 24 h, were 81.7 ± 6.0% in basal conditions and 90.2 ± 3.7% in the presence of VEGF, EGF, IGF-I, and FGF2 (n = 4, P = 0.066
Proliferation
HUVEC proliferation in basal conditions was 0.25 ± 0.18 O.D. and increased up to 1.85 ± 0.50 O.D. in the presence of VEGF, EGF, IGF-I, and FGF2 (n = 3–6,
Migration
Spontaneous migration of HUVEC was 25.2 ± 9.5 μm and increased by 87.8 ± 53.7%, up to 44.9 ± 13.4 μm in the presence of VEGF, EGF, IGF-I, and FGF2 in the bottom chamber (n = 17,
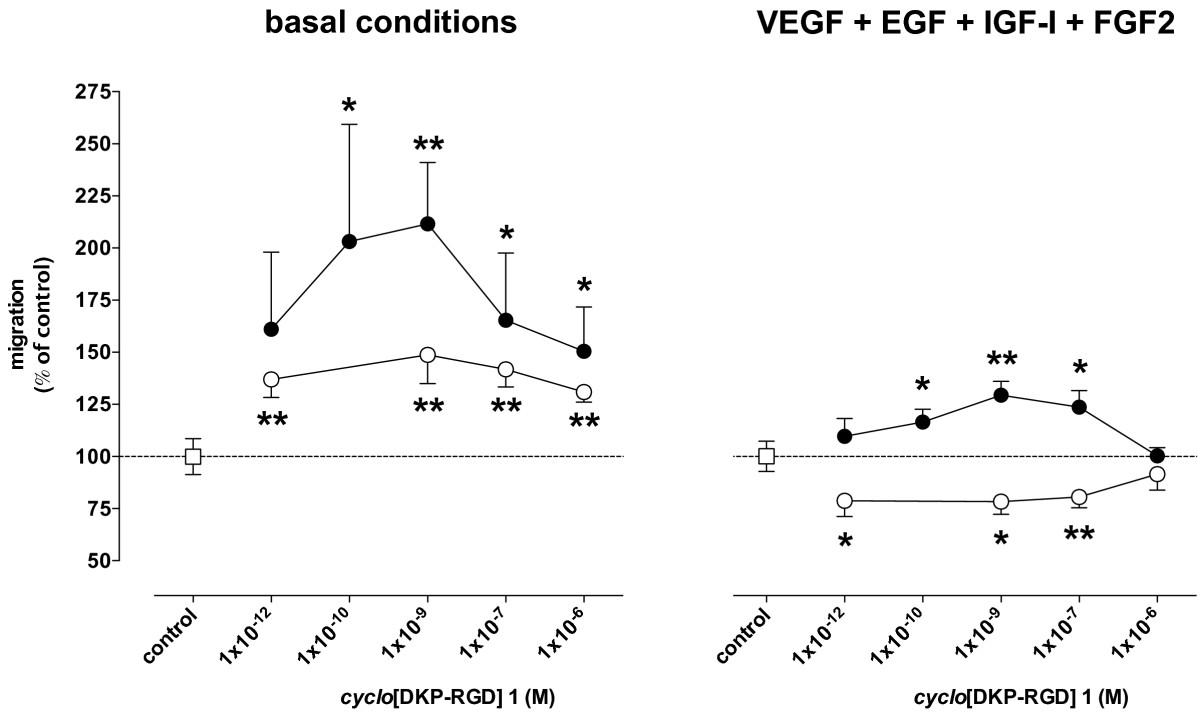
Figure 2
Figure 2 caption
Effect of[DKP-RGD] 1 on HUVEC migration in the Boyden chamber assay. Cells were placed in the top compartment. Empty circles: [DKP-RGD] 1 placed in the top compartment. Filled circles: [DKP-RGD] 1 placed in the bottom compartment. Data are means ± SEM of 5–17 separate experiments. * = P < 0.05 and ** = P < 0.01 vs respective control.
Angiogenesis
HUVEC under basal conditions did not show any significant network formation. Addition of VEGF, EGF, IGF-I, and FGF2 induced a significant network formation, which was even higher when cells were treated with IL-8 (Figure 3).
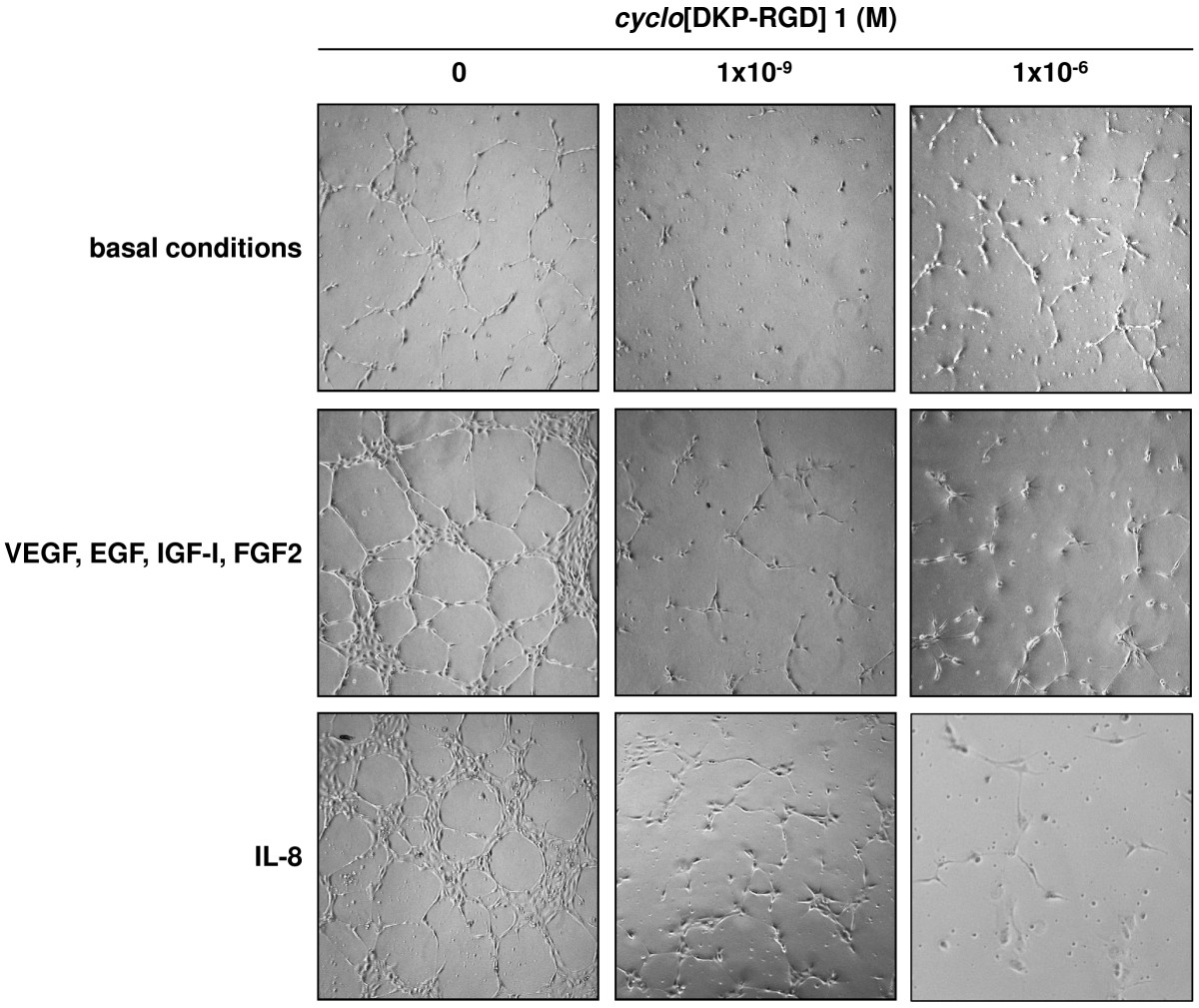
Figure 3
Figure 3 caption
Representative phase contrast photomicrographs of HUVEC plated on Matrigel in basal conditions or in the presence of VEGF, EGF, IGF-I, and FGF2 or IL-8, without and with[DKP-RGD] 1 at different concentrations.
Coincubation with
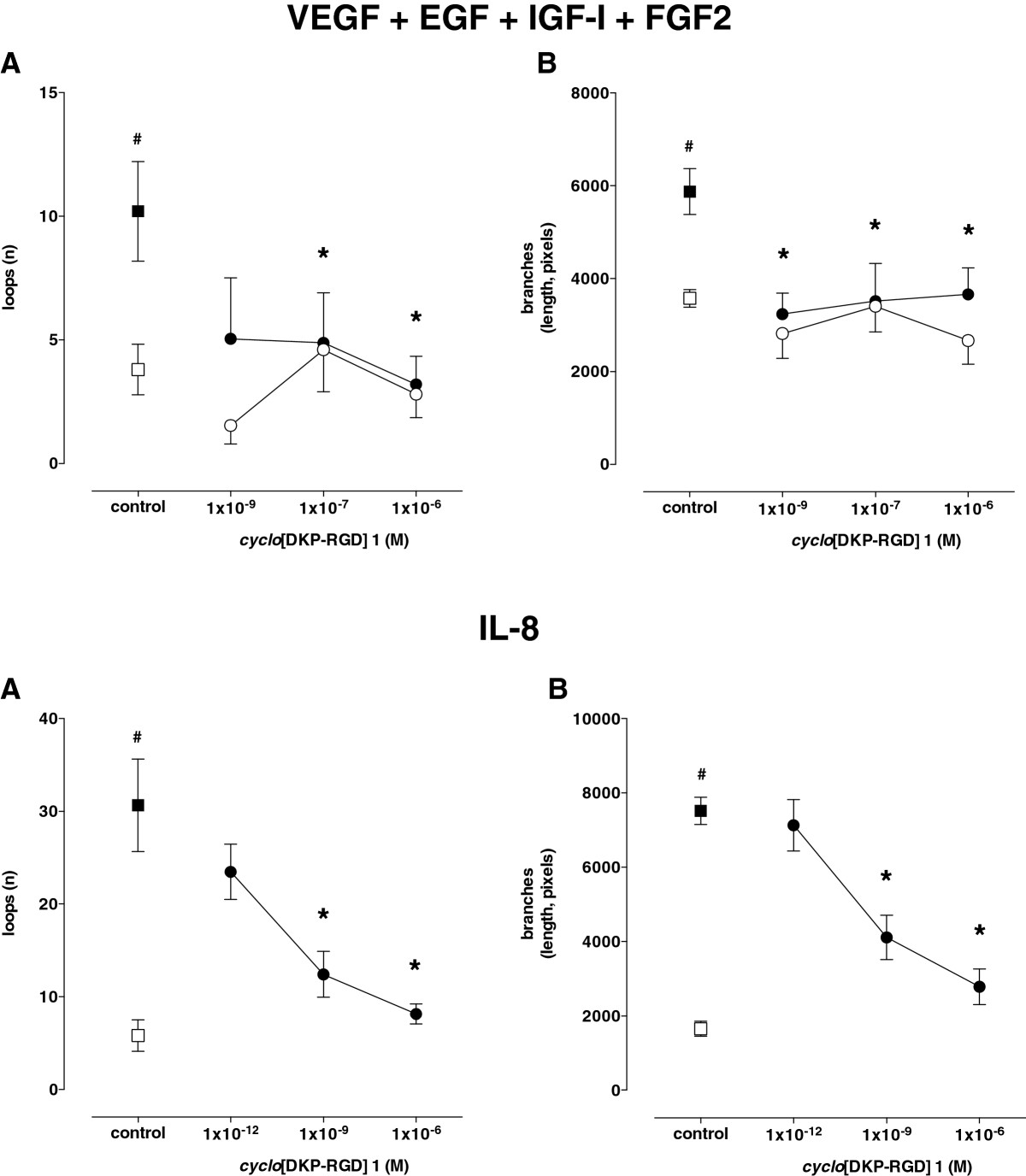
Figure 4
Figure 4 caption
Effect of[DKP-RGD] 1 on HUVEC angiogenesis induced by VEGF, EGF, IGF-I, and FGF2 (upper panels) or IL-8 (lower panels). Angiogenesis was evaluated as both number of loops (A) and length of branches (B). Empty symbols: basal conditions; filled symbols: stimulated conditions. Data are means ± SEM of 3–5 separate experiments. # = P < 0.01 vs basal conditions, * = P < 0.01 vs respective control.
Expression of mRNA for αv, β3 and β5integrin subunits
HUVEC expressed comparable amounts of the mRNA for αv, β3 and β5 integrin subunits in both basal conditions and after treatment with VEGF, EGF, IGF-I, and FGF2, and coincubation with 1×10−6 M
Table 2
Real time PCR analysis of the expression of mRNA for the integrin subunits αv, β3and β5in HUVEC cultured for 5 h in basal conditions and with VEGF, EGF, IGF, and FGF, alone (control) or in the presence of 1 μM
Subunit | control | + | ||
---|---|---|---|---|
A. Basal conditions | ||||
αv | 6.65 ± 6.41 | 6.59 ± 5.24 | 1.32 ± 0.57 | 0.944 |
β3 | 0.89 ± 0.87 | 0.91 ± 0.76 | 1.08 ± 0.27 | 0.928 |
β5 | 1.36 ± 1.18 | 1.52 ± 1.34 | 1.08 ± 0.07 | 0.225 |
B. With VEGF, EGF, IGF, and FGF | ||||
αv | 13.31 ± 12.80 | 15.09 ± 10.66 | 1.28 ± 0.42 | 0.494 |
β3 | 1.15 ± 1.07 | 1.41 ± 1.07 | 1.43 ± 0.35 | 0.288 |
β5 | 1.23 ± 1.25 | 1.31 ± 1.13 | 1.34 ± 0.46 | 0.461 |
Akt phosphorylation
Treatment of HUVEC with 1×10−6 M
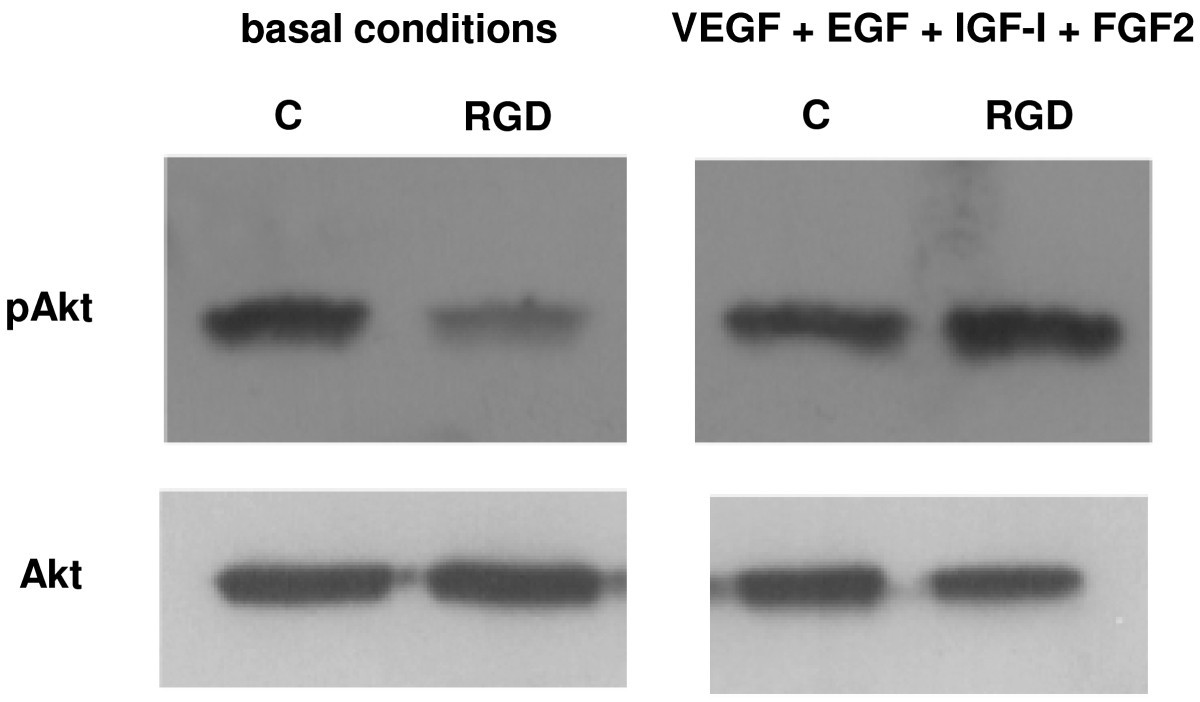
Figure 5
Figure 5 caption
Western blot analysis of Akt phosphorylation in HUVEC cultured for 5 h in basal conditions and with VEGF, EGF, IGF-I, and FGF2, alone (control, C) or in the presence of 1 μM[DKP-RGD] 1 (RGD). Data are from one representative of 3 separate experiments.
Discussion
HUVEC represent a valid
In our experiments, the effects of
Investigation of the specific mechanisms responsible for the antiangiogenic effects of
Disruption of integrin functions may possibly explain also the effects of
In our experiments,
In conclusion, the data of the present study show that the novel compound
Acknowledgements
This study was supported by a grant from Fondazione CARIPLO (Project RE-D DRUG TRAI-N 2010–1373:
Authors’ original submitted files for images
Below are the links to the authors’ original submitted files for images.
Authors’ original file for figure 1
Authors’ original file for figure 2
Authors’ original file for figure 3
Authors’ original file for figure 4
Authors’ original file for figure 5
References
- Mechanisms of angiogenesis and arteriogenesis. Nat Med. 2000;6:389-395.
- Peptide-based molecules in angiogenesis. Chem Biol Drug Des. 2006;67:115-126.
- IL-8 Directly enhanced endothelial cell survival, proliferation, and matrix metalloproteinases production and regulated angiogenesis. J Immunol. 2003;170:3369-3376.
- Signal transduction by cell adhesion receptor and the cytoskeleton: function of integrins, cadherins, selectins, and immunoglobulin-superfamily members. Annu Rev Pharmacol Toxicol. 2002;42:283-323.
- Integrins in angiogenesis: multitalented molecules in a balancing act. Cell Tissue Res. 2003;314:131-144.
- Ligand binding to integrins. J Biol Chem. 2000;275:21785-21788.
- Crystal structure of the extracellular segment of integrin alpha V beta 3 in complex with an Arg-Gly-Asp ligand. Science. 2002;296:151-155.
- Small molecule integrin antagonists in cancer therapy. Mini-Rev Med Chem. 2009;9:1439-1446.
- Ligands for mapping alphavbeta3-integrin expression in vivo. Acc Chem Res. 2009;42(7):969-980.
- Cilengitide: the first anti-angiogenic small molecule drug candidate. Design, synthesis and clinical evaluation. Anti-Cancer Agents Med Chem. 2010;10:753-768.
- Combination therapy of cilengitide with belotecan against experimental glioblastoma. Int J Cancer. 2013;133:749-756.
- First experience of 18 F-alfatide in lung cancer patients using a new lyophilized kit for rapid radiofluorination. J Nucl Med. 2013;54:691-698.
- Stimulation of tumor growth and angiogenesis by low concentrations of RGD-mimetic integrin inhibitors. Nat Med. 2009;15:392-400.
- Antagonistic VEGF variants engineered to simultaneously bind to and inhibit VEGFR2 and αvβ3 integrin. Proc Natl Acad Sci U S A. 2011;108:14067-14072.
- Cyclic RGD-peptidomimetics containing bifunctional diketopiperazine scaffolds as new potent integrin ligands. Chem Eur J. 2009;15:12184-12188.
- Cyclic RGD-peptidomimetics containing bifunctional diketopiperazine scaffolds as new potent integrin ligands. Chem Eur J. 2012;18:6195-6207.
- Role of Akt signaling in vascular homeostasis and angiogenesis. Circ Res. 2002;90:1243-1250.
- A small-molecule RDG-integrin antagonist inhibits cell adhesion, cell migration and induces anoikis in glioblastoma cells. International J of Oncology. 2013;42:83-92.
- Toll-like receptor 2 in promoting angiogenesis after acute ischemic injury. Int J Mol Med Mar. 2013;31:555-560.
- Cell-matrix adhesion in vascular development. J Thromb Haemost. 2007;1:32-40.
- Integrin alpha v beta 3 as a therapeutic target for blocking tumor-induced angiogenesis. Curr Drug Targets. 2003;4:123-131.
- Expression of integrins and adhesive properties of human endothelial cell line EA.hy 926. Cancer Genomics Proteomics. 2005;2:265-270.
- Cilengitide induces autophagy-mediated cell death in glioma cells. Neuro Oncol. 2011;13:857-865.
- Plaque neovascularization: defense mechanisms, betrayal, or a war in progress. Ann NY Acad Sci. 2012;1254:7-17.
- Synthesis and biological evaluation (in vitro and in vivo) of cyclic arginine-glycine-aspartate (RGD) peptidomimetic-paclitaxel conjugates targeting integrin αVβ3. J Med Chem. 2012;55:10460-10474.
- Integrin and growth factor receptor crosstalk. Circ Res. 2001;89:1104-1110.
- An Inhibitor of a Cell Adhesion Receptor Stimulates Cell Migration. Angew Chem Int Ed. 2010;49:7706-7709.
- The interleukin-8 pathway in cancer. Clin Cancer Res. 2008;14:6735-6741.
- Molecular pathways: fibroblast growth factor signaling: a new therapeutic opportunity in cancer. Clin Cancer Res. 2012;18:1855-1862.
- Targeting the vascular endothelial growth factor in hematologic malignancies. Eur J Haematol. 2012;89:373-384.
- Active antitumor immunity elicited by vaccine based on recombinant form of epidermal growth factor receptor. J Immunother. 2005;28:236-244.
- Angiogenesis in atherosclerosis: gathering evidence beyond speculation. Curr Opin Lipidol. 2006;17:548-555.