A clinically relevant model of stroke using aged rats
Vascular Cell. 2020;
Received: 17 August 2020 | Accepted: 13 October 2020 | Published: 16 November 2020
Vascular Cell ISSN: 2045-824X
Abstract
Diabetes, arterial hypertension and hypercholesterolemia are commonly described in elderly persons and are associated with higher risk of stroke. Therefore, it is of paramount importance to test the efficacy of cell therapies in an age-appropriate animal stroke model. Unfortunately, at the present time, animal models of stroke ignore age and comorbidities frequently associated with aging. Thus, numerous bench-to-bedside translation experiments evaluating neuroprotective strategies have been unsuccessful. In order to mimic more closely the clinical condition, we have established a model of ischemic stroke in aged rats. Using this model, we have showed that post-stroke angiogenesis is not impaired in the ischemic aged brain. Also, we have proved that stroke in aged rodent models reiterates closely the chain of pathological and clinical events described in stroke patients.
Keywords
stroke ageing comorbidities aged rat model angiogenesisIntroduction
Worldwide the incidence of cerebral ischemia is increasing due to unhealthy lifestyle, comorbidities and an increase in the number in the elderly population. Notably, individuals with a healthy lifestyle including physical, healthy diet, decent weight and moderate alcohol consumption have a lower risk of stroke than individuals with high-risk lifestyle. Stress and sedentary lifestyle associated with hypercholesterolemia, arterial hypertension, diabetes, obesity, are common in elderly persons and are associated with higher risk of stroke [2]. Moreover, simultaneous presence of vascular diabetic complications and associated comorbidities such as hypertension and chronic diabetes significantly increase the severity of ischemic cerebral damage [4].
Although advanced age is a risk factor for ischemic stroke, most of the experimental studies investigating this pathological condition have been conducted n young animals who unlike human patients recover rapidly. This would suggest that it is better to use aged rodents to test the efficacy of treatments aimed at improving behavioral recuperation after cerebral ischemia. In this context, we have established a model of ischemic stroke in aged rats. This model of ischemic stroke is presented below.
Middle cerebral artery occlusion in aged rats
In this study we have used aged male Sprague-Dawley rats (N= 56; 18–20 months of age; 520–600 g) kept under standard conditions of temperature and humidity in the Animal House Facility with free access to food and water. All procedures and protocols described in the study were performed in the Animal Facility of the University of Medicine and Pharmacy from Craiova, in accordance with the guidelines of Romanian Experimental Animals Protection Law. In addition, all the experiments were endorsed by our institution (Animal Experimentation Ethics Board of the University, 152/26112015).
Blood flow through the MCA was temporarily interrupted using the method described by us [3]. During surgery, anesthesia was maintained by spontaneous inhalation of 1 % to I.5% isoflurane, in a mixture of 75% nitrous oxide and 25% oxygen, through a specially designed mask. Body was controlled at 37 ± 0.2°C by a Homeothermic Blanket System (Harvard Apparatus, Edenbridge, Kent, U.K.). The anesthetized animals were placed in a supine position, and the tail artery was catheterized with PPSO (internal diameter = 0.58 mm). Cannulation of the tail artery enabled the measurement of blood pressure, arterial glucose levels and for determination of pH and blood gasses (Blutgassystem IL 1620, Instrumentation Laboratory, Munich; Omnican7 Balance, B. Braun, Melsungen Germany). Under a surgical microscope, the left and right common carotid arteries each were loosely encircled with a silicon thread to enable the temporary closure of these vessels. Subsequently, on the surgical table the rats were displayed in pronation with the head exposed on the right side. During the following step, a small segment of the skull above the MCA was removed at 3 mm rostral to the junction between the pars squamosa of the bone and the zygomatic arch. This procedure was done with the help of a microdrill (Fine Science Tools Heidelberg, Germany). The bone was thinned using the drill at low speed. To minimize friction-induced heating of the bone the drilling site was kept moisture with physiological saline. Finally, the bone flap was carefully removed using fine forceps and the dura opened using a fine needle.
At this point, the blood flow through the artery was completely blocked after MCA lifting performed with the help of a tungsten made hook elevated by a micromanipulator (Maerzhaeuser Precision Micro-Manipulator Systems, Fine Science Tools) [1]. Interrupting blood flow through the common carotid arteries by tightening the pre-positioned thread loops is essential for successful modelling of stroke. During MCA occlusion, the exposed cerebral cortex was kept moist using artificial cerebrospinal fluid to keep (Liquicheck, Spinal Fluid Control, BioRad; Germany). After ninety minutes, the hook was removed and the common carotid arteries were opened, allowing for brain reperfusion. Finally, the skin was sutured and the animals returned to their cages. To minimize post-surgery pain and suffering animals were given buprenorphine.
After a 3 to 60 days survival time, the rats were anesthetized with 3% isoflurane in 75% nitrous oxide 25% oxygen and perfused with buffered saline followed by buffered, 4% freshly depolymerized paraformaldehyde. The brains were removed, post fixed in 4% buffered paraformaldehyde for 24 hours, cryoprotected in 20% sucrose prepared in 10 mmol/L phosphate-buffered saline (PBS), flash-frozen in isopentane, and stored at -70°C until sectioning.
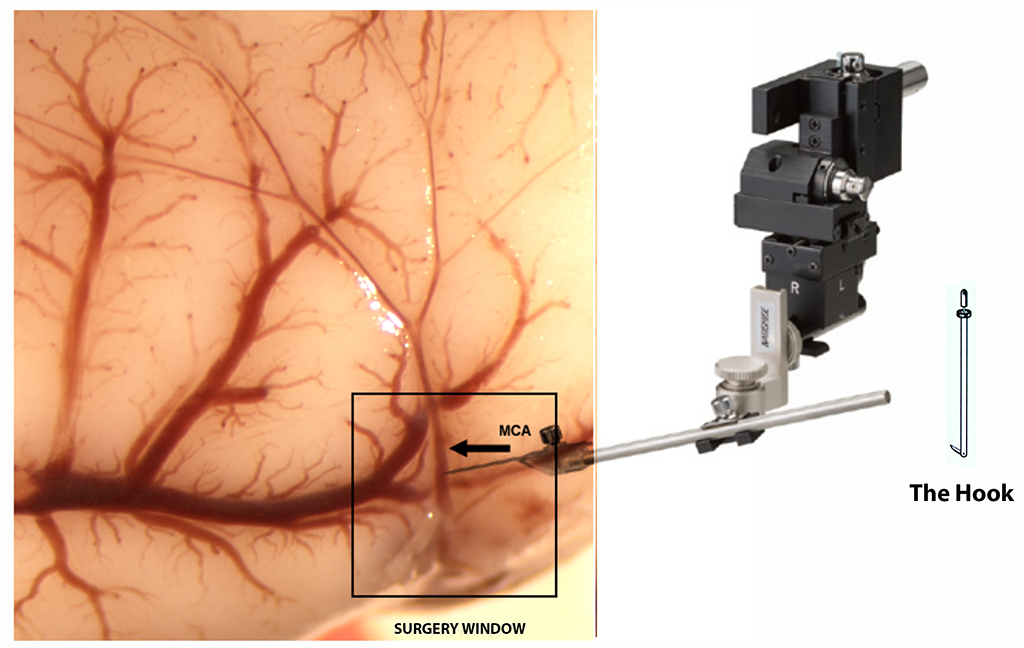
Figure 1
Figure 1
Schematic drawing of the experimental procedure to induce stroke in aged rats.
Immunohistochemistry of aged rat tissue
The experimental subjects were administered bromodeoxyuridine (BrdU; 50 mg/kg body weight, i.p.) daily for seven days. This facilitated the identification on newly formed blood vessels. Free floating sections were incubated first with a mouse anti-rat endothelial cell antigen (RECA) (1:200, abcam, UK) followed by a donkey-anti-rabbit-FITC 1:3000, (Dianova, Hamburg, Germany). Subsequently, the tissue sections were fixed in 4% paraformaldehyde, followed by BrdU exposure. For BrdU detection, free-floating sections were incubated in 2M HCl at 37◦C for 30min. To neutralize solution acidity, sections were washed several times with 0.1M borate buffer (pH 8.5) until pH was 7.2. Then, sections were incubated in blocking solution containing 10% goat serum, 0.2% gelatin, 0.1-0.3% Triton X-100, in PBS overnight at 4◦C, followed by rat anti-BrdU antibody (1:2000, AbD Serotec, UK) at 4◦C for 48 h. BrdU-positive cells were visualized by incubating either with Cy3-conjugated donkey anti-rat IgG (H+L) (1:3000).
Post-stroke angiogenesis in aged rats
Hypoxia powerfully stimulates angiogenesis. During the activation phase of angiogenesis, endothelial cells (ECs) and fibroblasts migrate into extracellular spaces, proliferate and release cytokines and angiogenic chemokines like CXCL1, CXCL12/CXCR4 and vascular endothelial growth factor (VEGF), which in turn activate circulating endothelial progenitor cells (EPCs), which eventually integrate into tube structures and differentiate into mature EC. Thus, at 3 days after stroke, BrdU-labeled cells invade the peri-infarcted area [2A, arrowheads]. RECA-labeled blood vessels are also detectable [2A, arrow]. However, at 7 days post-stroke the blood vessel became tortuous and leaky allowing BrdU-labelled cells, presumably of hematogenous origin, to enter the infarcted area in great numbers [2B, arrowheads]. In regions far away from the damaged cortex, in the subventricular zone, we detected numerous BrdU-labelled, newly formed blood vessels in the ventricular wall [2C, arrowheads]. In the close proximity, there were old, RECA-labelled blood vessel [2C, arrow]. This finding reveals that the ependymal cell layer is highly dynamic and involves continuous synthesis of blood vessels. The presence of BrdU-labelled blood vessels in the contralateral hemisphere lends support to this hypothesis [2D]. However, after a stage characterized by significant ECs and EPCs proliferation, these newly formed cells interact dynamically creating complex three-dimensional capillary-like tubular structures [1]. Indeed, at 28 days after stroke numerous RECA/BrdU double-labelled blood vessels, ie newly formed blood vessels, were seen in the peri-infarcted area of the aged brains [3A, arrow]. The infarcted area is isolated from the brain environment by a scar tissue, a region considered by many to be refractory to axonal growth. However, our results show that this region is highly penetrated by a network of newly formed blood vessels [3B, arrow] which could supply the scar tissue with blood and nutrients. Beyond the scar tissue, within a region which we labelled as an “islet of regeneration” was characterized by strong angiogenesis [~~FIGREF~~3C, arrow] suggesting that advanced age is not a limitation factor for post-stroke angiogenesis. The presence of BrdU-labelled blood vessels in the contralateral hemisphere lends support to this hypothesis [3D, inset]. However, in young animals we noted more numerous blood vessels but no overlapping of BrdU-labelled with RECA-labelled capillaries [3E, arrows].
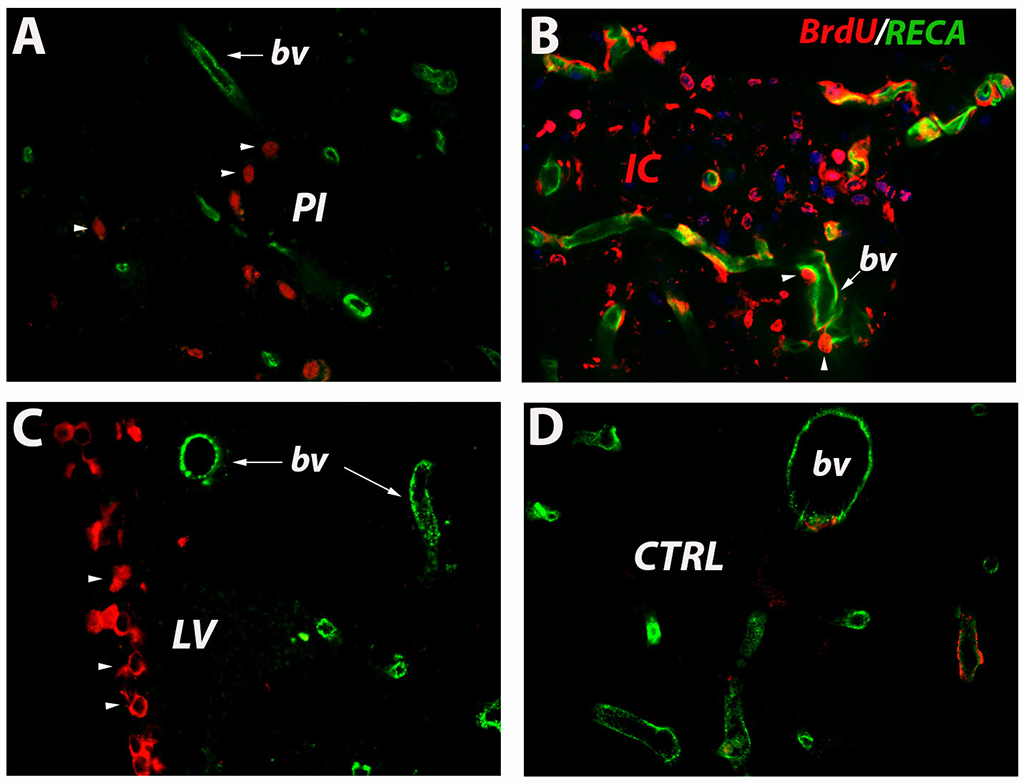
Figure 2
Figure 2
Angiogenesis early after stroke. At 3 days after stroke, BrdU-labeled cells invade the peri-infarcted area (A, arrowheads). RECA-labeled blood vessels are also detectable (A, arrow). However, at 7 days post-stroke the blood vessel became tortuous and leaky allowing BrdU-labelled cells, presumably of hematogenous origin, to enter the infarcted area in great numbers (B, arrowheads). Newly formed capillaries were also noted in the subventricular wall (C, arrowheads). In the close proximity, there were old, RECA-labelled blood vessel (C, arrow). New endothelial cells were also noted in the contralateral hemisphere (D).
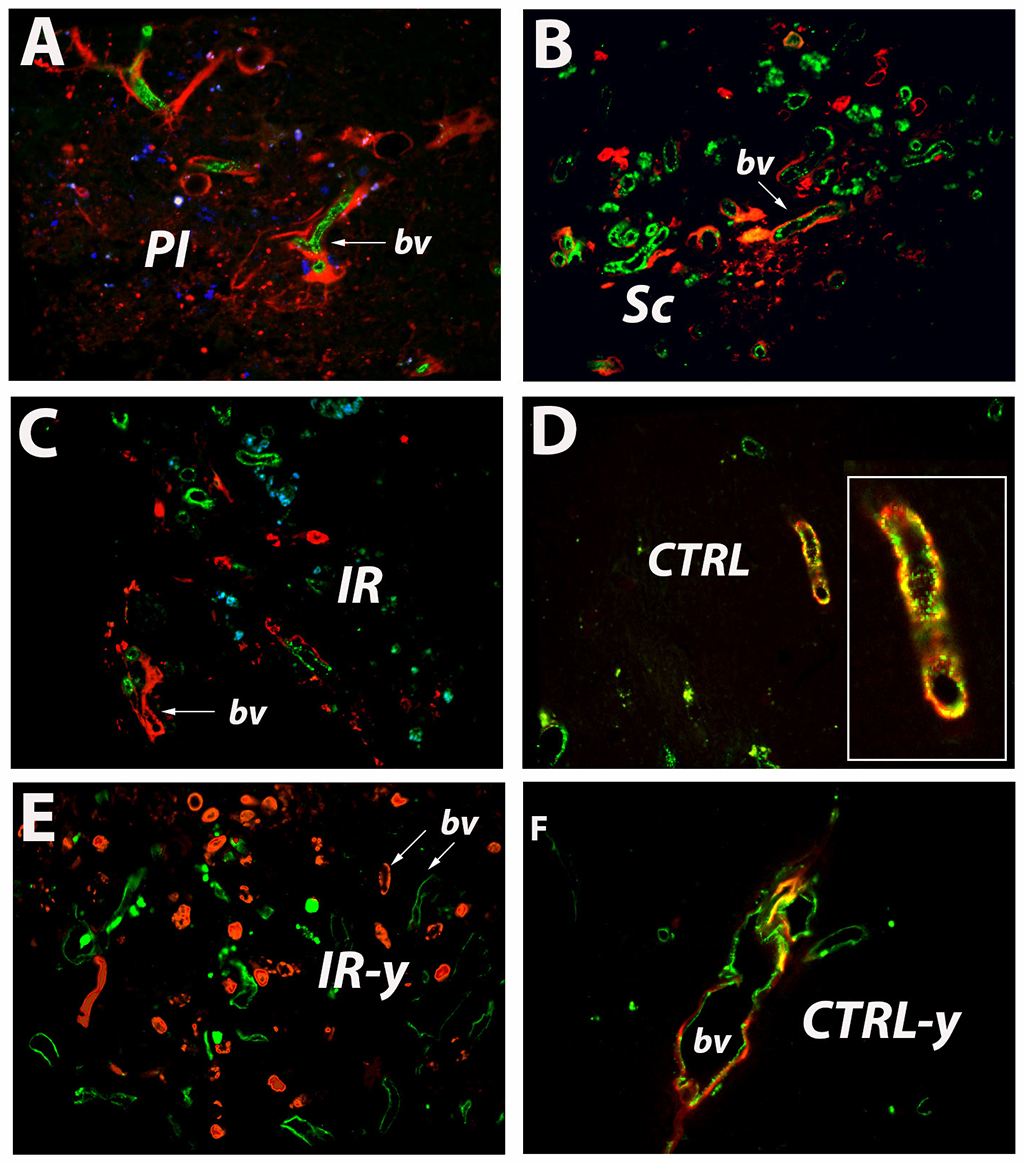
Figure 3
Figure 3
Angiogenesis during the recovery phase. At 28 days after stroke numerous newly formed blood vessels, were seen in the peri-infarcted area of the aged brains (A, arrow). The a scar tissue is highly penetrated by a network of newly formed blood vessels (B, arrow). Beyond the scar tissue there is angiogenesis, too (C, arrow). WE also noted BrdU-labelled capillaries vessels in the contralateral hemisphere (D, inset; F). However, in young animals we noted more numerous blood vessels but no overlapping of BrdU-labelled with RECA-labelled capillaries (E, arrows).
The cerebral vascular system in adults is considered to be steady under normal physiological conditions. However, recently we have shown that in uninjured adult rat brain there is a continuous remodeling of the brain vasculature showed by the incorporation of BrdU into the nuclei of endothelial cells lining the lumen of existing blood vessels.
Following ischemic injury, blood vessels in remote areas relative to the infarct core and in the contralateral non-lesioned cortex, showed co-labelled BrdU/RECA+ endothelial cells shortly after the BrdU injection. This is a remarkable finding indicating that endothelial cells could have a bone marrow origin. Most likely, these cells probably entered the brain from the circulation via leptomeningeal blood vessels. In addition, cells located in the close proximity to collagen IV-labelled basement membrane, In the peri-infarcted area, displayed double labelling with BrdU/ prolyl 4-hydroxylase beta, a marker of proliferating endothelial cells. Therefore, we believe that proliferating vascular cells could be bone-marrow derived as well as resulting from disintegration of vascular wall [5]. In addition, the patchy distribution of newly incorporated endothelial cells in mature blood vessels of the adult rat brain is highly suggestive of random incorporation of the new endothelial cells into the mature cerebral blood vessels [5]. Finally, the presence of BrdU-labelled blood vessels in the contralateral hemisphere [3F] lends support to this hypothesis.
Conclusions
The mortality rate in the aged animal model of stroke is higher (30%) than in young animals. However, post-stroke angiogenesis is not impaired in the old ischemic brain. Overall, the aged murine ischemic model of stroke is a reliable approach for the study of this condition mimicking closely the clinical and pathological stages seen in human patients.
Acknowledgements
This work was supported by the EU Framework Programme for Research and Innovation, Horizont 2020, project number 667302 to APW, and UEFISCDI, project numbers PN-III-P4-ID-PCE-2016-0340 to DMH; PN-III-P2-2.1-PED-2016-1013 and PN-III-P4-ID-PCE-2016-0215 to APW.
Original submitted files for images
Below are the links to the authors’ original submitted files for images.
Original image file for Figure 1
Original image file for Figure 2
Original image file for Figure 3
References
- Transcriptomics of post-stroke angiogenesis in the aged brain. Front Aging Neurosci. 2014;6:44-. doi:10.3389/fnagi.2014.00044
- Ageing as a risk factor for cerebral ischemia: Underlying mechanisms and therapy in animal models and in the clinic. Mech Ageing Dev. 2020;190:111312-. doi:10.1016/j.mad.2020.111312
- beta-Amyloid precursor protein and ss-amyloid peptide immunoreactivity in the rat brain after middle cerebral artery occlusion: effect of age. Stroke. 1998;29(10):2196-2202. doi:10.1161/01.str.29.10.2196
- Inducing stroke in aged, hypertensive, diabetic rats. J Cereb Blood Flow Metab. 2010;30(4):729-33. doi:10.1038/jcbfm.2009.273
- Vasculature Remodeling in a Rat Model of Cerebral Ischemia. The Fate of the BrdU-Labeled Cells Prior to Stroke. Front Neurol. 2018;9:1014-. doi:10.3389/fneur.2018.01014