Angiogenesis-osteogenesis coupling: a key element in bone physiology and regeneration
Vascular Cell. 2021;
Received: 20 September 2021 | Accepted: 11 October 2021 | Published: 20 December 2021
Vascular Cell ISSN: 2045-824X
Abstract
Bone represents a well vascularized structure which is remodelled and renewed continuously. It consists of osteoblasts, osteoclasts and osteocytes which have a precise role in the context of endochondral and intramembranous ossification. While the link between the bone tissue, bone marrow and the haematopoiesis is crucial for the generation of progenitor bone forming cells, recent research indicates that bone physiology as well as bone healing, repair and regeneration is directly dependent on bone angiogenesis. Experimental research suggest that angiogenesis and osteogenesis are directly coupled through a mechanism in which type H endothelial cells have a role of paramount importance. Apart from type H endothelial cells, other molecular elements such as HIF and Notch as well as CD31-endomucin capillaries and miR-497∼195 endothelial clusters may have a significant role in angiogenesis-osteogenesis coupling. The number of type H endothelial cells decreases significantly in elderly and is paralleled by a significant drop in the supply of progenitor cells. This would explain the bone loss and the decreased bone regeneration potential seen in elderly patients. Overall, it is suggested that endothelial cells and bone angiogenesis would represent therapeutic targets in various pathological conditions characterized by bone loss and impaired regeneration.
Keywords
Angiogenesis Osteogenesis coupling VEGF FGF PIGF Osteoblasts Enchondral ossification Intramembranous ossificationIntroduction
The bone tissue is highly vascularized and remodelled throughout life to remain intact and consists of several different cell types; osteoblasts, osteocytes and osteoclasts cells [1]. Osteoclasts formed by the fusion of mononuclear progenitors of the macrophage-monocyte cell will promote bone resorption while in parallel, maintaining the blood calcium homeostasis [1, 2]. Conversely, osteoblasts derived from local mesenchymal cells residing along bone the surface, actively synthesise bone while the osteocytes represent inactive resting osteoblasts [1,3] All these cells have a specific functional role in the context of intramembranous and endochondral ossification, complex processes which required the participation of several types of cells and growth factors [1,2,3].
Intramembranous ossification involves the direct differentiation of mesenchymal stem cells into osteoblasts and is the main mechanism leading to a development of flat bones such as the clavicle. However, endochondral ossification represents replacement of a cartilaginous structure into a bone structure and is described in long bones such as the tibia and the femur [3].
Bone formation also persists throughout life to alter the geometry of bones, so that they are sufficient to withstand changes in mechanical loading (modelling), to replace aged or damaged bone (remodelling) or to fill-in fractures. In both modes of bone formation and at all stages of life, skeletal vasculature plays a significant role as will supply not only nutritive and growth factors but also progenitor stem cells creating a dynamic link between the hematopoietic microenvironment, bone marrow and bone tissue. At the present time, it is known that these progenitor stem cells have a role of paramount importance providing an adequate number of elements which will differentiate into bone, cartilaginous or adipose cells. This is observed during bone growth and development as well as during bone sculpting and remodelling [4].
All these data would suggest that bone physiology as well as bone repair, healing and regeneration would depend on bone vascularization and a local angiogenesis which frequently have been overlooked in experimental studies evaluating various methodologies for bone regeneration. However, recent research suggests that the key element in bone regeneration would be represented by the endothelial cells in the context of angiogenesis-osteogenesis coupling.
a. General aspects of angiogenesis
A well developed and functional vascular network is of paramount importance for an optimal embryogenesis, tissue healing, regeneration, and reproduction as the vascular network provides oxygen and nutrients to all tissue structures maintaining overall fluid homeostasis and oxygenation of all biological structures. In the embryonic stage, we can distinguish two different types of processes that lead to formation of embryonic blood vessels, vasculogenesis and angiogenesis. Vasculogenesis consist in mesoderm differentiation as well as the appearance of hemangioblasts derived-primitive tubular blood vessels with formation of internal primitive vascular plexuses and yolk sac circulation [5, 6].
Anyway, to achieve full potential functionality these newly formed vascular structures require remodelling, which is achieved through angiogenesis, a complex process which includes interaction of numerous cells, growth factors and cytokines and is realized via two different mechanisms: the endothelial cell sprouting and the intussusceptive microvascular growth. While the endothelial sprouting is made possible by endothelial cell migration, proliferation and tube formation, intussusceptive microvascular growth represents the appearance of tissue folds and columns ultimately separating the previously formed vascular lumens as well as formation of new vessels by in situ loop formation in the wall of veins [6].
In the early process of embryogenesis, the first endothelial forming elements, the hemangioblasts are differentiated by fibroblast growth factor with initial formation of blood islands of the yolk sac [5]. Research conducted in patients with single-cell bone marrow transplant indicated the presence of adult hemangioblasts suggesting that angiogenesis repair is present also in adult population [7]. Hemangioblasts which are seen in the bone marrow and other non-hematopoietic sites express the same immunohistochemical profile such as CD34 and ACC133. The most important modulator for these hemangioblasts is represented by vascular endothelial cell growth factor (VEGF) and its receptor, the receptor tyrosine kinase flk-1 which activate a paracrine system stimulating angiogenesis [5]. Anyway, Schatteman et al (2004) suggest that hemangioblasts represent an important source of endothelial cells for physiological angiogenesis as well as for numerous pathological conditions being a major element in tissue repair. In addition, another source of progenitor cells for endothelial differentiation is represented by the circulating monocytes which are activated in their turn by hemangioblasts [7]. Ultimately, the newly formed endothelial cells will expand all over the vascular lining interfering with fundamental physiological and physiopathological processes such as vascularization in normal conditions, after stroke and cancer, in homeostasis, vascular exchanges with interstitial space, fibrinolysis and coagulation, maintaining an optimal vascular tonus under certain requirements as well as cell migration and activation [5].
However, the most important modulator of physiological and pathological angiogenesis especially during embryogenesis and skeletal growth and development is the vascular endothelial growth factor (VEGF) which stimulates cell division and migration meantime, facilitating vascular wall permeability [8, 9]. Studies conducted in a mice model, have indicated that VEGF-A promote in mice robust formation of several vascular structures such as mother vessels, glomeruloid bodies and daughter capillaries [10].
VEGF has a significant role in numerous musculoskeletal diseases, for example promoting fracture healing or promoting rheumatoid arthritis pathogenesis [11]. Also, several molecules with similar action with the initial VEGF have been discovered and at the present time all of these are described as a VEGF family group including VEGF-A, VEGF-B, VEGF-C, VEGF-D, viral VEGF-E, VEGF-F as well as placenta growth factor (PIGF) and endocrine-derived vascular endothelial growth factor (EG-VEGF) [9]. Matsumoto and Ema (2014) have reported that VEGF-A pathway is the most important during in-utero development, modulating most of the endothelial cell functions including migration and growth, vascular wall permeability. VEGF-A is a robust pro-angiogenic factor in the post-natal period modulating the release of numerous paracrine factors by the endothelium subsequently improving the rate of regeneration [12].
VEGF exerts its action via several types of tyrosine kinase receptors such as VEGFR-1, also known as Fms-like tyrosine kinase 1 (Flt-1), which is receptor for VEGF-A, VEGF-B as well as placental growth factor (PIGF) expressed by both endothelial and some cancer cells [9, 13]. VEGFR-2 also known as kinase insert domain receptor (KDR) or fetal liver kinase 1 (Flk-1) seems to be the most important receptor mediating the actions of VEGF being a marker for endothelial cells differentiation. VEGFR-2 also modulates pathological angiogenesis in cancer suggesting that it may be an important therapeutic target [14]. However, its synthesis is modulated by another VEGF receptor, called VEGFR-3 or Fms-like tyrosine kinase 4 (Flt-4). This factor modulates lymphangiogenesis after VEGF-C and BEGF-D binding. However, it also promotes sprouting of newly formed capillaries and overall vascular network development. Research conducted in genetically modified mice with VEGFR-3 gene deletion have shown that missing this receptor results endothelial leakage. Studies conducted in this genetically modified mouse model, have also indicated an overexpression of VEGFR-2 associated with a reduced amount of the cellular tightening molecule named endothelial cadherin, at the cell-cell junctions in both postnatal animals as well as in endothelial cultures suggesting that VEGFR-3 would inhibit VEGFR-2 synthesis and VEGF/VEGFR2, overall preventing vascular leakage [15].
Recent experimental studies conducted in a murine model indicates that fibroblast growth factor (FGF) exerts its pro-angiogenic role through c-myc modulation which subsequently modulates production of glycolytic enzyme hexokinase 2 (HK2). Ultimately, an optimal level of HK2 will sustain endothelial cells migration and proliferation suggesting that FGF-dependent endothelial glycolysis is a process of paramount importance in angiogenesis [16].
In addition, numerous authors have reported that endothelial cells differentiation and angiogenesis could be stimulated by mechanical pressure, remodelling and numerous other factors and molecules such as interleukin-8 (IL-8), tumour necrosis factor-alpha, transforming growth factor-alpha (TGF-alpha), transforming growth factor-beta (TGF-beta), hepatocyte growth factor/scatter factor (HGF/SF), platelet endothelial cell adhesion molecule (PECAM-31), Vascular Endothelial-cadherin and ETS-transcription domain factor [17, 5, 18].
Also, experimental research has indicated that a new type of tyrosine kinases my interfere with angiogenesis. Apart from being present on endothelial cells, Tie-1 tyrosine kinase and Tie-2 tyrosine kinase may be identified in association with macrophages and are of paramount importance for blood vessels maturation [19]. Sato et al (1995) have demonstrated in a murine model that subjects deficient in Tie-1 have leaking vascular endothelium and develop haemorrhage [20]. Tie-2 exert its action through binding to Angiopoietin 1-4 (Ang-1-4). More specifically, Ang-1 promotes Tie-2 phosphorylation followed by an improvement of the endothelial cells’ barrier [21]. No ligand has yet been identified for Tie-1 which can interfere with Tie-2 pathway. Anyway, Ang-1 is a critical player in the process of vessel maturation mediating migration, adhesion, and survival of endothelial cells. Overall, its action promotes creation of robust vessels and has a protective role in several important pathological conditions including diabetic neovascularization, diabetic nephropathy, oxygen induced-retinopathy, sepsis, and cardiac allograft transplantations [22]. Interestingly, Ang-2 has an interesting action. By itself it induces apoptosis and vascular regression, but it also has a synergic effect with VEGF increasing new blood formation [19].
Remarkably, the hypoxia-inducible factor 1 (HIF-1) is a very important modulator of angiogenesis promoting angiogenesis synergistically along with the angiopoietins pathways, in concert with VEGF and placental growth factor (PIGF) [23].
Apart from all these molecular factors, morphological components of the blood vessels are very important for functionality and development of a vascular network. The most important morphological element is represented by the endothelial cells and recent studies suggest that these cells are in fact coordinating centres for regeneration and healing processes preventing disease. They are also part of the stem cell niches and have a role of paramount importance in neurogenesis, haematopoiesis, and bone formation [24]. The vascular stem cells niches include a wide range of growth factors called angiocrine factors actively involved in all organ regeneration stages, induction, specification, and patterning. The synthesis of angiocrine factors is increased after any type of tissue damage but the molecular factors involved in synthesis and release of these angiocrine molecules, in time and space, are not completely elucidated [25]. On the outer surface of the vessels these are covered by perivascular-mural cells called pericytes or vascular smooth muscle cells. The ontogeny of these cells is poorly understood. If the number of pericyte is not adequate the vessels will become dilated and haemorrhagic, and these patients will develop edema and diabetic retinopathy [26].
Considering all these, we may conclude that development of an efficient vascular network requires fully functional endothelial cells in terms of their mobility, proliferation, and cell differentiation. However, the intricate mechanisms of vessel assembly are poorly understood. Also, the molecular pathways by which endothelial cell sprouting is stopped is not known nor are the specific pathways and molecules involved in modulating the vascular tube formation. Anyway, it is believed that all of these may be modulated by VEGF family group and other related factors with synergistic action [27].
b. General aspects of bone angiogenesis
Bone, like any other tissue, requires vascularisation, the local vascular network having an important paracrine function, releasing numerous growth factors, and interacting with several types of cells. In bone angiogenesis, the endothelial cells and overall, the vascular structures have a role of paramount importance for skeletal development and growth, bone remodelling, and healing processes. During bone remodelling as osteoclasts move forward and reabsorb bone, blood vessels will follow in proximity. Blood vessels also serve as a structural template for bone development and bring the key elements for bone homeostasis into the osteogenic microenvironment, such as minerals, growth factors and osteogenic progenitor cells [3].
A study by Maes et al (2010) confirmed the strong relationship between angiogenesis and osteogenesis, indicating that entry of osteoblast precursors into developing bones is intimately related to invasion by blood vessels. More specifically, the authors revealed that precursors exhibit a perivascular localisation and directly associate with invading blood vessels, ultimately suggesting the possibility of a coupled movement process involving mechanisms that are specific to early cells in the osteoblast lineage [28].
In the process of endochondral ossification, the origin of trabecular osteoblasts is not known but numerous osteoblasts are seen in the area next to the avascular cartilage. Studies conducted in transgenic mice have suggested that osterix-labelled osteoblasts precursors located in the perichondrium would differentiate into trabecular osteoblasts as well as osteocytes and bone stromal cells. This process has been described as a typical translocation. However, some of the osteoblast precursors have also been identified in very close proximity of the invading blood vessels, displaying a pericyte-like topography. Remarkably, a similar pattern has been recorded in bone fractures but in this case the outer mature osteoblasts did not show perivascular topography. These cells will remain in the cortex of developing bones [28].
c. Endochondral ossification and angiogenesis
Endochondral ossification is described in long bones. The process of endochondral ossification represents the transformation of an embryonic cartilage structure in a fully functional bone. In the early stages the chondrocyte will proliferate and hypertrophy and ultimately will become apoptotic and die (Figure 1). However, they will also manufacture extracellular matrix which will be penetrated by blood capillaries that supply the structure with nutritive elements as well as growth factors. In addition, monocyte-macrophages and osteoclasts appear as the osteoblasts start manufacturing and laying down bone on the cartilage [29, 30].
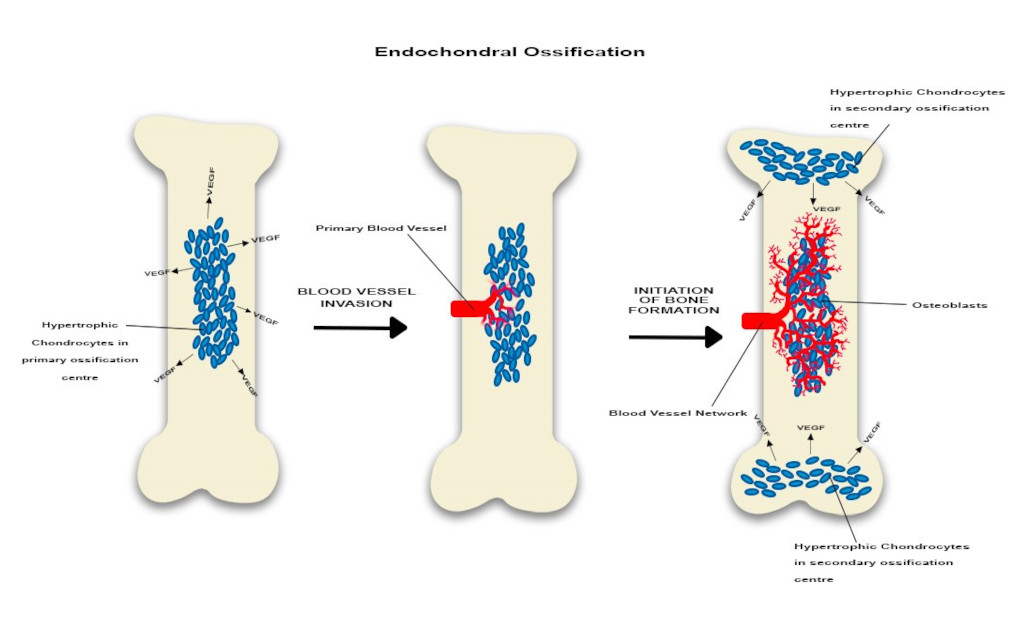
Figure 1
Figure 1
Endochondral ossification is characterized initially by the mesenchyme derived chondrocytes which are present the primary ossification centre. Some will hypertrophy and will secrete VEGF which promotes local angiogenesis. Subsequently the capillaries will expand towards both epiphyses. Ultimately, in the stage of active bone formation, the vascular network is well represented and will promote the development of the secondary ossification centre. VEGF is continuously released by hypertrophic chondrocytes located in the secondary ossification centres and osteoblasts are clearly identified. Overall, this suggests angiogenesis-osteogenesis coupling.
Endochondral ossification is facilitated and enhanced by endochondral angiogenesis. However, endochondral angiogenesis is turned on and amplified by a group of chondrocytes located in the primary ossification centre area which would hypertrophy initially. Then these cells will synthesize pro-angiogenic molecules promoting local formation of new blood capillaries. In addition, the local osteoprogenitor cells will also secrete pro-angiogenic factors enhancing angiogenesis. These newly formed blood vessels will create an extensive vascular network surrounding the chondrogenic cells. Interestingly, as the vascular network develops, ossification becomes more obvious [29, 30]. Ossification and vessels growth will also develop along a longitudinal axis. In long bones, the hypertrophic chondrocytes located in the growth plates promote development of diaphyseal and metaphyseal capillary network which will expand near the epiphyseal chondrocytes located at the extremity of the long bones. Subsequently, this process would determine formation of the secondary ossification centres which will complete the ossification process [31].
The action of chondrocytes is modulated by systemic factors such as thyroid factors, growth hormone (GH) and locally released factors such as: fibroblast growth factor (FGF), parathyroid hormone-related peptide (PTH-rP), and Indian hedgehog factor [30]. It seems that the process of endochondral ossification is modulated by the Wnt/beta-catenin molecular pathway which promotes an optimal development, inhibiting in the early stages of biological development, the chondrocyte hypertrophy while later, enhancing cellular hypertrophy as well as ossification. This suggest that this molecular pathway is of paramount importance for growth plate development as well as defining cartilage boundary definition and endochondral ossification [32]. In addition, some authors have reported that the chondrocyte function is also modulated by extracellular factors such as MEF2C, Runx2 and Sox 9 which would interfere directly chondrocyte gene expression. However, the action of these factors takes place in concert with expansion of the vascular network [30].
Langen et al (2017) indicate that the endothelial vasculature of the post-natal long bones includes a special type of endothelial cells named E. These cells sustain the osteoblasts cells. If the relevant endothelial integrins β1 are abnormal or missing, the subject shows both deficient bone growth as well as endothelial/vascular differentiation [33]. More importantly, Filipowska et al. (2017) suggests that specialised endothelial cells which line the blood vessels found in bone, namely, type H and L, are permeable to growth factors stimulating differentiation of bone precursor cells involved in bone regeneration. Also, research work conducted by other groups confirmed that activation of type H endothelial cells promote osteogenesis [24, 31]. Studies performed in a murine model, have reported that although new blood vessel formation and osteogenesis are linked, a new type of capillary which seems to be involved in this coupling would produce specific molecules facilitating angiogenesis-osteogenesis coupling as well as maintaining an optimal amount of osteoprogenitor cells [31]. According to this theory, the most important element, the type H endothelial cells would modulate the functionality of the local blood vessels while providing a niche for the osteoprogenitor cells. The development of H type blood vessels is enhanced by HIF and Notch molecular pathways as well as estrogen. The decline in the HIF and Notch pathways as well as in the estrogen level is associated with a decrease in type H capillaries and osteoprogenitor cells resulting in impairment of bone formation and osteoporosis [31]. As the number of type H endothelial cells is age-dependent, a gradual decrease over time leads to age-related loss of bone precursor cells, explaining the diminished regeneration potential of the bone observed in the elderly [3, 31].
Xie et al (2014) have described another type of blood vessels which facilitates the angiogenesis-osteogenesis coupling. These vessels are positive for CD31 and endomucin. However, studies conducted in a murine model have demonstrated that platelet-derived growth factor-BB (PDGF-BB) depleted mice have specifically low trabecular and cortical bone mass as well as less CD31-endomucin positive blood vessels compared with the normal subjects. However, administration of PDGF-BB increases the number of osteoclasts but also it will increase the number of CD31-endomucin capillaries promoting bone formation [34]. Recent research suggests that CD31-endomucin blood vessels are also modulated by the G protein-coupled receptor kinase 2 interacting protein-1 (GIT1). This is a scaffold protein of paramount importance in bone healing and remodelling which promotes angiogenesis-osteogenesis coupling [35]. Studies performed in GIT1 knock out mice show a significant decrease in trabecular bone mass and CD31-endomucin blood vessels. In all these subjects the concentration of PDGF-BB was significantly decreased [35]. Recent experimental studies conducted in a murine model suggests that CD31-endomucin blood vessels are also positive for miR-497∼195 cluster. A decrease in this marker is associated with a lower bone mass. However, in transgenic subjects characterized by an increase in miR-497∼195 in the endothelial cells there is less bone loss, as this cluster facilitates HIF and Notch functionality. In elderly subjects, intravenous injection of aptamer-agomiR-195 promotes formation of bone and CD31-endomucin blood vessels [36]. This could represent a better therapeutic approach for those with loss of bone mass.
d. Intramembranous ossification and angiogenesis
Intramembranous ossification is described in flat bones and includes several events characterized by expression of chondrogenic and osteogenic markers including bone morphogenic proteins which modulate transformation of neural crest-derived mesenchyme to bone-forming cells, osteoblats [37]. Compared with endochondral ossification, this process does not require a cartilaginous stage, but robust angiogenesis is sine qua non condition (Figure 2)
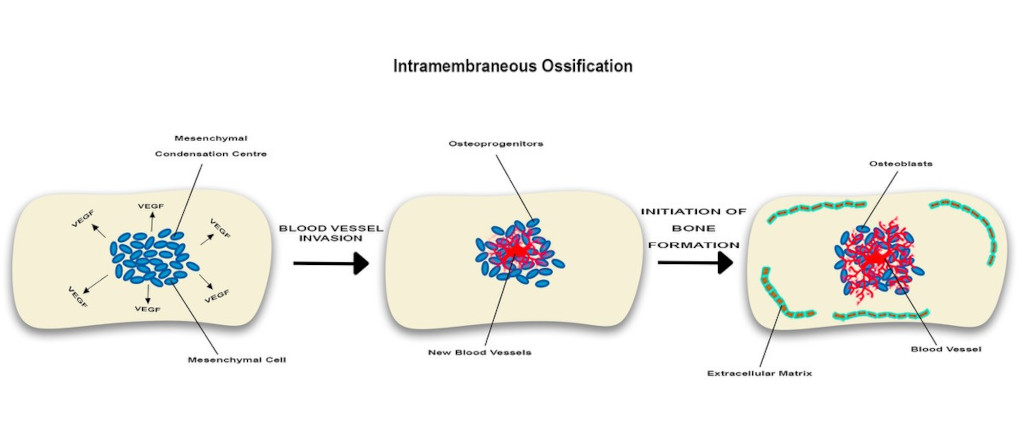
Figure 2
Figure 2
In intramembranous angiogenesis while no chondrocytes are seen, some mesenchymal cells will form mesenchymal condensation centres which will produce VEGF promoting local angiogenesis. Near newly formed blood vessels, some of these mesenchymal cells will subsequently become bone-forming elements, osteoblasts. These cells will manufacture extracellular matrix facilitating local development of a vascular network which in turn would enhance osteogenesis. Overall, this suggests angiogenesis-osteogenesis coupling.
Blood vessel formation during intramembranous ossification was first described by Thompson et al. (1989) who examined intramembranous bone formation in the developing frontal bone of chick embryos and demonstrated the stages of blood vessel invasion. Shortly before the initial ossification of the frontal bone, capillaries move into the thin avascular layer of loose mesenchyme, which surrounds the mesenchymal condensation centre, where mesenchymal cells secrete VEGF, attracting endothelial cells. The capillaries then invade the mesenchymal condensation centre at or nearby the site of initial ossification. Bone then undergoes mineralisation and associates with the internal and external extensive network of capillaries. As bone expansion continues, a further cascade of vascular invasion and ossification continues where osteoblasts participate in mineralisation, laying down new bone at the site of large capillaries [38].
Conclusions
In conclusion, angiogenesis is of paramount importance for embryonic and postnatal bone development providing the necessary oxygen, nutrients as well as the osteoprogenitor cells which would provide the basis for continuous bone regeneration. There is clear evidence that angiogenesis and osteogenesis are linked but the intricate mechanisms and factors involved in this process are not fully understood. However, it seems that in this setting the endothelial cells have a role of paramount importance controlling bone regeneration potential by supporting the bone forming elements, the osteoblasts and supplying the required amount of osteoprogenitor cells. Recent research has revealed crucial physiological functions are performed by new elements such as endothelial H cells as well as HIF and Notch molecular pathways, CD31-endomucin capillaries and miR-497∼195 endothelial clusters. However, the number of type H endothelial cells decreases significantly with aging and is associated with a lower level of bone progenitor cells [6]. All of these would explain the bone loss and decreased bone regeneration potential seen in elderly subjects with various pathologies, suggesting that endothelial cells and angiogenesis would represent therapeutic targets requiring activation or upregulation. In this context, more experimental studies are urgently needed to elucidate all the factors involved in bone angiogenesis and osteogenesis-angiogenesis coupling.
Original submitted files for images
Below are the links to the authors’ original submitted files for images.
Original image file for Figure 1
Original image file for Figure 2
References
- Bone vascularization in normal and disease conditions. Front Endocrinol (Lausanne). 2013;4:106-. doi:10.3389/fendo.2013.00106
- An overview of bone cells and their regulating factors of differentiation. Malays J Med Sci. 2008;15(1):4-12.
- The role of vasculature in bone development, regeneration and proper systemic functioning. Angiogenesis. 2007;20(3):291-302. doi:10.1007/s10456-017-9541-1
- Bone marrow stromal stem cells: nature, biology, and potential applications. Stem Cells. 2001;19(3):180-192. doi:10.1634/stemcells.19-3-180
- Vasculogenesis. Annu Rev Cell Dev Biol. 1995;11:73-91. doi:10.1146/annurev.cb.11.110195.000445
- Vasculogenesis and angiogenesis. Cancer Treat Res. 2004;117:3-32. doi:10.1007/978-1-4419-8871-3_1
- Hemangioblasts, angioblasts, and adult endothelial cell progenitors. Anat Rec A Discov Mol Cell Evol Biol. 2004;276(1):13-21. doi:10.1002/ar.a.10131
- Vascular endothelial growth factor and the regulation of angiogenesis. Recent Prog Horm Res. 2000;55:15-35.
- Vascular endothelial growth factor (VEGF) - key factor in normal and pathological angiogenesis. Rom J Morphol Embryol. 2018;59(2):455-467.
- VEGF-A(164/165) and PlGF: roles in angiogenesis and arteriogenesis. Trends Cardiovasc Med. 2003;13(5):169-175. doi:10.1016/s1050-1738(03)00056-2
- From the cradle to the clinic: VEGF in developmental, physiological, and pathological angiogenesis. Birth Defects Res C Embryo Today. 2003;69(4):363-374. doi:10.1002/bdrc.10024
- Roles of VEGF-A signalling in development, regeneration, and tumours. J Biochem. 2014;156(1):1-10. doi:10.1093/jb/mvu031
- Therapeutic implication of vascular endothelial growth factor receptor-1 (VEGFR-1) targeting in cancer cells and tumor microenvironment by competitive and non-competitive inhibitors. Pharmacol Res. 2018;106:97-107. doi:10.1016/j.phrs.2018.08.023
- Vascular endothelial growth factor receptor-2 in breast cancer. Biochim Biophys Acta. 2010;1806(1):108-121. doi:10.1016/j.bbcan.2010.04.004
- VEGFR3 Modulates Vascular Permeability by Controlling VEGF/VEGFR2 Signaling. Circ Res. 2017;120(9):1414-1425. doi:10.1161/CIRCRESAHA.116.310477
- FGF-dependent metabolic control of vascular development. Nature. 2017;545(7653):224-228. doi:10.1038/nature22322
- Angiogenesis. J Biol Chem. 1992;267(16):10931-10934.
- Mechanisms of angiogenesis. Nature. 1997;386(6626):671-674. doi:10.1038/386671a0
- Angiopoietins in angiogenesis. Cancer Lett. 2013;328(1):18-26. doi:10.1016/j.canlet.2012.08.018
- Distinct roles of the receptor tyrosine kinases Tie-1 and Tie-2 in blood vessel formation. Nature. 1995;376(6535):70-74. doi:10.1038/376070a0
- Tie1 controls angiopoietin function in vascular remodeling and inflammation. J Clin Invest. 2016;126(9):3495-3510. doi:10.1172/JCI84923
- Therapeutic targeting of the angiopoietin-TIE pathway. Nat Rev Drug Discov. 2017;16(9):635-661. doi:10.1038/nrd.2016.278
- Hypoxia-Inducible Factor-1 in Physiological and Pathophysiological Angiogenesis: Applications and Therapies. Biomed Res Int. 2015;2015:549412-. doi:10.1155/2015/549412
- Regulation of tissue morphogenesis by endothelial cell-derived signals. Trends Cell Biol. 2015;25(3):148-157. doi:10.1016/j.tcb.2014.11.007
- Angiocrine functions of organ-specific endothelial cells. Nature. 2016;529(7586):316-325. doi:10.1038/nature17040
- The role of pericytes in blood-vessel formation and maintenance. Neuro Oncol. 2005;7(4):452-464. doi:10.1215/S1152851705000232
- Molecular control of endothelial cell behaviour during blood vessel morphogenesis. Nat Rev Mol Cell Biol. 2011;12(9):551-564. doi:10.1038/nrm3176
- Osteoblast precursors, but not mature osteoblasts, move into developing and fractured bones along with invading blood vessels. Dev Cell. 2010;19(2):329-344. doi:10.1016/j.devcel.2010.07.010
- Developmental regulation of the growth plate. Nature. 2003;423(6937):332-336. doi:10.1038/nature01657
- Endochondral ossification: how cartilage is converted into bone in the developing skeleton. Int J Biochem Cell Biol. 2008;40(1):46-62. doi:10.1016/j.biocel.2007.06.009
- Coupling of angiogenesis and osteogenesis by a specific vessel subtype in bone. Nature. 2014;507(7492):323-328. doi:10.1038/nature13145
- Developmental regulation of Wnt/beta-catenin signals is required for growth plate assembly, cartilage integrity, and endochondral ossification. J Biol Chem. 2005;280(19):19185-19195. doi:10.1074/jbc.M414275200
- Cell-matrix signals specify bone endothelial cells during developmental osteogenesis. Nat Cell Biol. 2017;19(3):189-201. doi:10.1038/ncb3476
- PDGF-BB secreted by preosteoclasts induces angiogenesis during coupling with osteogenesis. Nat Med. 2014;20(11):1270-1278. doi:10.1038/nm.3668
- GIT1 is critical for formation of the CD31hiEmcnhi vessel subtype in coupling osteogenesis with angiogenesis via modulating preosteoclasts secretion of PDGF-BB. Bone. 2019;122:218-230. doi:10.1016/j.bone.2019.03.006
- MiR-497∼195 cluster regulates angiogenesis during coupling with osteogenesis by maintaining endothelial Notch and HIF-1α activity. Nat Commun. 2017;8:16003-. doi:10.1038/ncomms16003
- Regulation of skeletogenic differentiation in cranial dermal bone. Development. 2007;134(17):3133-3144. doi:10.1242/dev.002709
- Intramembranous osteogenesis and angiogenesis in the chick embryo. J Anat. 1989;166:55-65.